When he asked his father what it was, he was told it was a direct line to a vast underwater lake with an unlimited volume of water. Solomon was fascinated by the idea which raised many questions for him: Where did it come from? How long has it been there? And how did his father, who admittedly had “immense practical knowledge,” according to Solomon, know that?
“Well, he was wrong. Sort of,” says Solomon who as a child may have been imagining an underwater lake that you could waterski on. “If you dug a hole, it's not like an underground cavern or something. It was in a different context,” he concedes. But the groundwater is there, and it’s massive: ten Lake Powells’ worth below just the Salt Lake Valley.
But that “different context” of his father’s claim of an underground lake, was something Solomon, recently recognized with the Hydrogeology Division of the Geological Society of America’s prestigious O. E. Meinzer Award, would learn about during the next three decades. Most recently, Solomon, who in September was also elected Fellow by the American Geophysical Union, has been using environmental tracers to evaluate groundwater flow and solute transport processes in local- to regional-scale aquifers.
In particular, the esteemed hydrogeologist has developed the use of dissolved gases including Helium-3 (3He), Chlorofluorocarbons (CFCs) and Sulfur hexafluoride (SF6) to evaluate groundwater travel times, location and rates of recharge and the sustainability of groundwater resources. In fact, at the U, the former department chair who was recently announced as interim chair of the Department of Geology & Geophysics, constructed and operates one of only a few labs in the world that measures noble gases in groundwater. His research results have been documented in more than 125 journal articles, book chapters, and technical reports.
Recharge rates and residence times
So, why not just start pumping out those ten Lake Powells of freshwater standing below the Salt Lake Valley and other regions where sub-surface aquifers are brimming with the stuff?
The short answer to that is, well . . . we’ve already tried doing that and ill-advisedly, we’re still doing that. This is true not just in the American West, but across the globe from Africa to the Negev desert in Israel and from South America to the great Ogallala aquifer which underlies America’s famed breadbasket, an area of approximately 174,000 square miles in portions of eight states.
The second short answer is that we are courting ecological and human disaster if we don’t look closely at recharge rates and residence times — the time it takes for those aquifers to fill up as they have over hundreds, thousands even millions of years — of depleted volumes. What Solomon and his colleagues are bringing to the table — the water table, as it were — is more and more sensitive and complicated measurements of a startlingly complex system.
The third short answer, related back to Utah and the depleted Great Salt Lake now in a state of crisis, is that the good snow years we had in 2023 and 2024 did not refill the sub-surface bathtub of the Great Basin and certainly will not “fix” the problem of water scarcity. “We could pump this system,” Solomon says in a guarded tone, “we could fill the Great Salt Lake up easily … Okay? But only once. And then we might have to wait a few hundred years or a few thousand years to fill that system back up. That's the caveat.”
The Sandbox
Solomon’s lab in the Sutton Building looks like the sandbox of a dimly lighted playground straight out of a B-movie: an impressive array of copper tubes and steam punk-styled oxidized baubles, huge humming spectrometers, beakers and refrigerators, plunging samples to 10 degrees Kelvin.
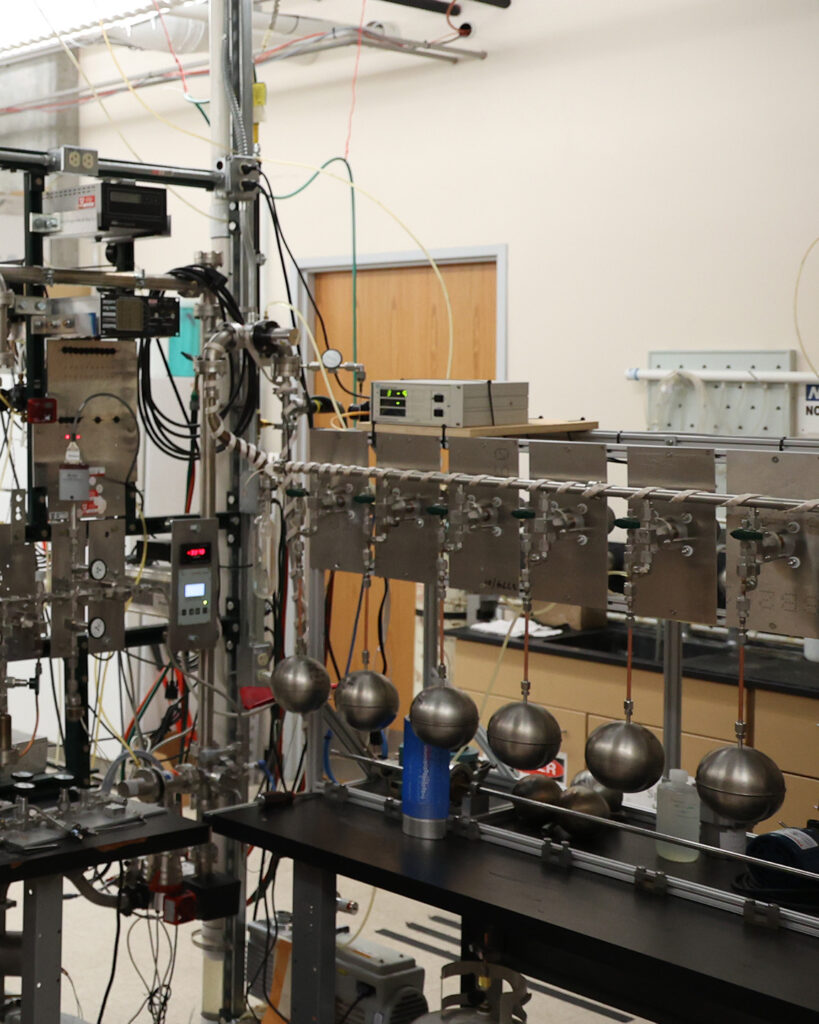
Copper tubes that suck out the gases that are dissolved in the water specimen from which measurements of 3He are secured. Credit: Todd Anderson
“Minus 263 degrees,” exclaims Solomon over the humming of equipment. “That's very cold, you know. And we have to do that to separate the noble gases, one from another.” Cryogenically separating these gasses is required to measure one thing at a time, and it is technology and equipment that also can break, frequently.
“Imagine that you are cooling to minus 260 degrees and then warming to plus 30 or 40 degrees and you were doing that hundreds of times a day,” he says, two of his lab group Emily Larsen and Will Mace looking on. (“Will’s over there nervous that I'm gonna break something,” quips Solomon as he continues the tour.) “It's always temperature swings and so forth. And then just, you know, just cooling to the insulation that's required to be able to cool to that temperature.”
It’s all part of the process of dating groundwater by measuring tritium, a radioactive isotope of hydrogen that decays in a half-life of 12 years or so, to 3He, a rare, stable and non-radioactive isotope of helium. In the soup of it all is Larsen, preparing specimens by eliminating extraneous gases and sealing them up, placing them on a shelf for six weeks and letting tritium decay to the stable noble gas of 3He which is then measured.
In addition to measuring tritium, the team deploys a procedure in nearby copper tubes that sucks out the gases that are dissolved in the water specimen from which measurements of 3He are taken. It is the ratio of tritium to 3He3 that measures how long the water has been in the ground (its age).
The Solomon lab’s findings paint a much more complex … and sobering picture of how depleted groundwater, overwhelmingly the largest volume of fresh water that's available on Earth, gets re-charged and how long it can take. “I'm an engineer,” says Solomon, “so I'm always looking for solutions, but you can't look for a decent solution until you really understand the problem.”
So, while that massive volume of water under the Salt Lake Valley does in fact exist, the rates of which water is recharged to the subsurface and moves through the subsurface of that reservoir is small and exceedingly difficult to measure due to variability that is “mind boggling.”
That limited transfer is largely related both to climate and the amount of precipitation. But it’s also related to geology, “how well rocks and sediments are able to transmit water,” says Solomon referring to permeability, a property of the Earth’s soil that first motivated his work. Of late, there is an accumulating literature about the age of water, another metric that impacts our understanding of transfer rates and might lead to new water management policies and the “solutions” that the engineer in Solomon is constantly scanning the substrate for.
Why study the age of water? “If you can measure how long it took for that water to go from where it got recharged, to where you're collecting it, or to where it's discharging, now you have a means, a different sort of method to evaluate groundwater flow systems.”
One thing is for certain in the world of hydrogeology: without even knowing it, you can easily use more groundwater than is being sustainably recharged. And it’s happening right now across the globe.
A covenant with water
Talk at any length with Solomon about one of the defining issues of our day — water depletion on a warming globe — and you learn that there is no quick fix. To put a finer point on it, maybe “fixing” a system, as if taking some kind of plumber’s wrench to it, is decidedly not the way forward, the wrong word altogether. Perhaps instead we as a society should be looking at making a covenant or promise with water — a play on the book title by medical doctor-turned-novelist Abraham Verghese — and then honoring it.
Solomon recounts recent work he has been doing in Nebraska that is one of eight states reliant on the now shrinking Ogallala aquifer. “They do something called ‘tanking.’ They go get a big farmer's watering tank that they use for their livestock. They throw it in the creek and get some paddles and probably a case of beer and they float down the Middle Loop River. And it's great fun.” Some of that river water, he explains, is a few hundred years to 8,000 years old. “On average,” he says, “they're floating on water that first fell from the sky 3,000 years ago,” the opening salvo of the Bronze Age.
The misperception of water and its ways isn’t just rampant in Nebraska, or Utah . . . it’s global. We more commonly think of lakes and rivers as our primary water source when they are fractional compared to groundwater. And yet we behave as if that groundwater is static, infinitely replaceable in a span of time to our liking, and easily measurable. Solomon and his colleagues are doing no less than shifting the paradigm on that and in a sense almost personifying groundwater as complex, dynamic and as elusive as your grandchild. (And equally nigh unto impossible to quantify and “successfully” navigate.)
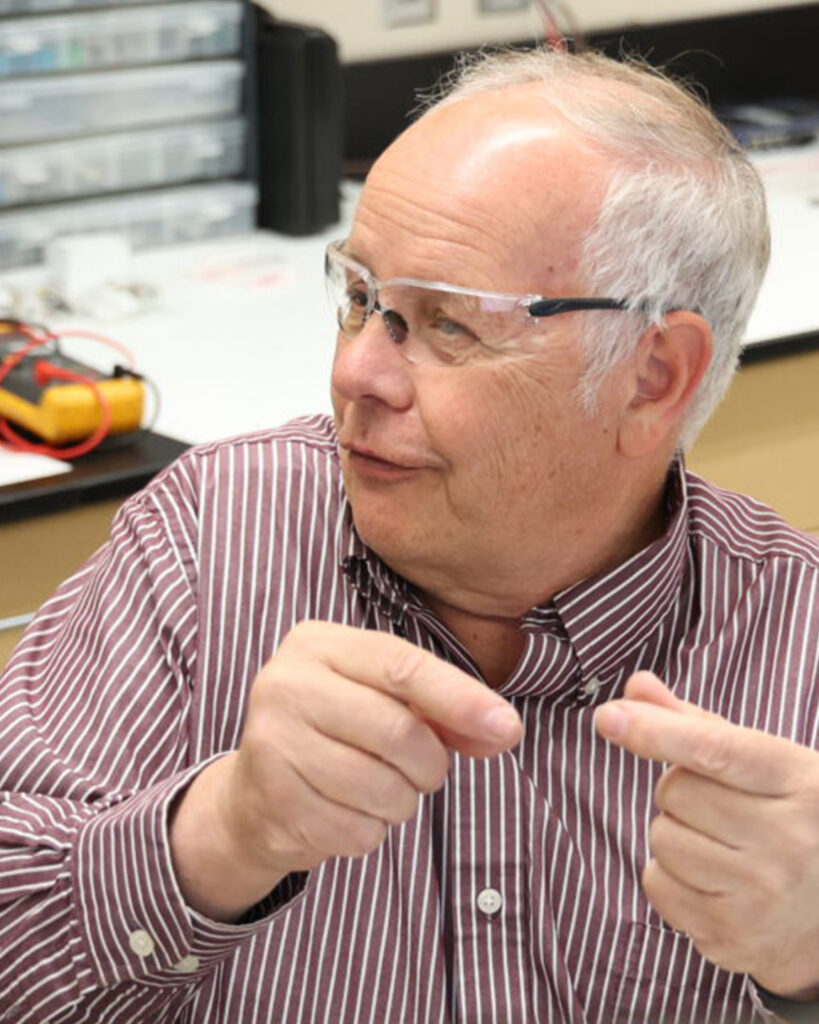
Kip Solomon explaining how noble gases are measured. Credit: Todd Anderson
In hydrology, water management lags theory by at least 30 years, says Solomon. “It takes a long time when new concepts emerge. It takes a long time to finally get that trickled [down] into practice.” That the whole hydrologic system has memory is the shift in thinking. “We are, especially practitioners, just starting to come to grips with the fact that, that we can't just look at one year of snow and precipitation and so forth.” For example, colleague Paul Brooks and Solomon have been doing some work looking at streams coming out of Red Butte Canyon in the foothills just south of the University of Utah campus. “That water recharged fifty years ago — recharge meaning [that’s when] it got into the ground. When it fell as precipitation.” The takeaway here in a community that prides itself on being hyper-aware of snowfall, snowmelt and precipitation is that it isn't enough to look at the annual amount of precipitation.
“There's memory in the system because the subsurface can store lots of water but releases it slowly."
In his work Solomon, who holds the Frank Brown Presidential Chair, travels a lot, having been on virtually every continent and advises other countries through the United Nations about out to understand groundwater systems. Recently, in the desert country of Morocco, he says, “they know that they're over-pumping their groundwater by a billion cubic meters a year. And, you know, they're trying to figure out what to do about it. But among other things, I advise them to look at the age of the water and use that to help refine their models of groundwater flow. My worry is that what they think is a billion might be 10 billion, because right now, their models do not benefit from having kind of age-data.”
The Meinzer Award
If water, groundwater in particular, is such that we should make a covenant with it to understand, respect it—including its age—and manage it as if it’s a sacred, intimate partner, then research in the vein of Solomon’s is key to that. He and other of his ilk are attempting to understand rates of recharge not just by making physical measurements, but by looking at permeability, age of water and movement of it along a flow path. It’s an infinitely more robust approach worthy of the complex subject of water.
“I think that's why I'm probably getting the Meinzer Award,” Solomon says without a milliliter of hubris.
A first-generation college student, Solomon epitomizes the best that science and engineering has to offer the curious and the adventurous. Though always interested in geology and that mysterious pipe disappearing into the ground on his father’s lot, he knew he would have to “make a living” and became an engineer in the College of Mines and Earth Sciences. But like the subject that has been his life’s work his career has wended its way—from its descent as precipitation, it’s absorption into the substrate as groundwater, it’s recharge and discharge. Now “recharged” in the College of Science as a professor of geology and geophysics (as well as a second round as department chair) he has embraced all of it: geology, geophysics and inorganic chemistry right into the cutting-edge science of isotopes.
But he has never strayed far from his engineering roots and the practical applications of knowledge. If anyone has the authority to make policy and practical management suggestions related to groundwater, it is Kip Solomon.