Sci Fri: Cosmic Ray Burst
Around 30 years ago, scientists in Utah were monitoring the skies for cosmic rays when they detected a surprising particle. It struck the atmosphere with much more energy than they had previously seen—enough energy to cause the researchers to dub it the “Oh My God Particle.”
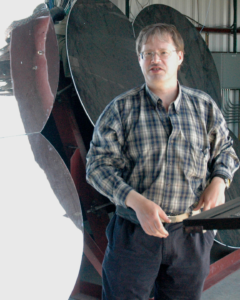
John N. Matthews of the U's Department of Astronomy and Physics, standing beside large telescope mirrors at the Telescope Array Project's florescence detector station just outside the Drum Mountains, Millard County, Utah. Photo by Joe Bauman, May 25, 2013. Banner Photo above: The surface detector array of the Telescope Array experiment, deployed by helicopter. Credit: Institute For Cosmic Ray Research, University Of Tokyo
Over the years, a collaboration of researchers in Utah and Japan has detected other powerful rays—about 30 a year—but none that rival the OMG. In 2021, however, a second particle was detected. It was only slightly less powerful than OMG, but still many times more powerful than can be created on Earth. That 2021 particle was named “Amaterasu,” after a sun goddess from the Japanese Shinto religion. The researchers described their observations in a recent issue of the journal Science.
The researchers believe the particle must have come from relatively nearby, cosmically speaking, as otherwise it would likely have collided with something in space and lost its energy. However, when they tried to trace the particle back to its origin in space, they were unsuccessful. Both the OMG particle and the new Amaterasu particle seem to have come from empty regions of space, with no violent events or massive structures to create them.
Dr. John Matthews, a research professor in physics and astronomy and manager of the Cosmic Ray Physics Program at the University of Utah, joins Ira to talk about cosmic rays, how they’re detected, and the challenges of finding the origin of particles like Amaterasu.