Answering fundamental questions about the chemistry that drives variability in air pollution formation & impacts climate.
There may not be a lot in common with Salt Lake City and Forsyth, GA, population 4,239, but Monroe County’s seat–other than being home to the county’s only high school–does have a small community theater with the same name as one of Salt Lake City’s most notable venues: “The Rose.”
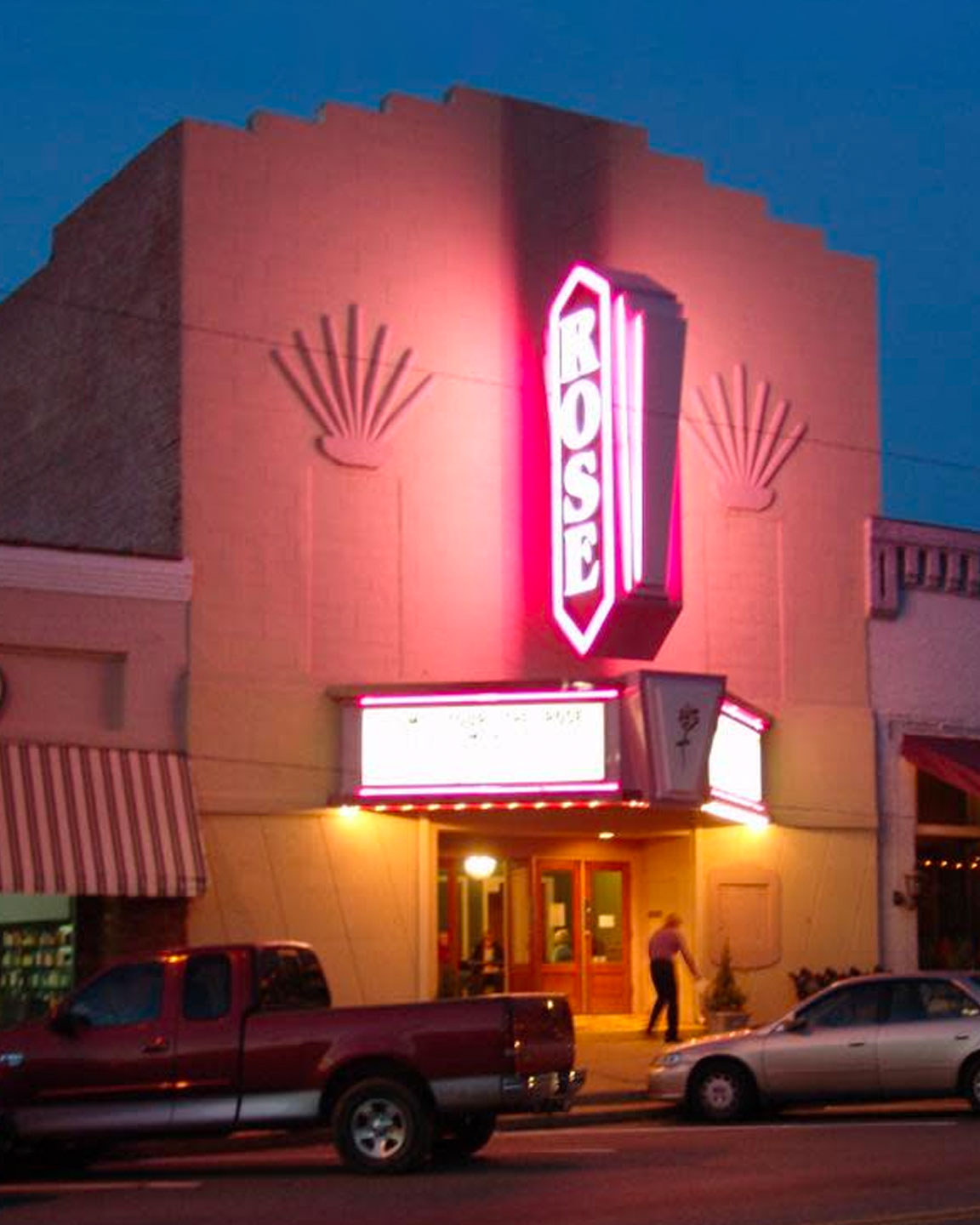
The Rose Theater
In Forsyth, the Rose Theater appears to stage family-friendly shows: “Four Weddings and An Elvis” closes in February. Later, this November 11th, there’s a single-night engagement that looks like an annual outing, “Hometown Gospel Sing.”
The theatre located on Forsyth’s town square is emblematic of the small-town life in which Jessica Haskins grew up before winning a full-ride, need-based scholarship to Massachusetts Institute of Technology (MIT). And her move from rural Georgia to the east coast megalopolis was shocking for reasons other than just the differences in weather and academic rigor. "It was a punch in the face” says Haskins, “coming to MIT, and realizing that the experience of most Black Americans outside the southeast, particularly in STEM fields, is one where they often find themselves the only non-white person in the room.”
In fact, Haskins' time at Mary Persons High School was much more diverse than MIT, ranked at the time by the Princeton Review as the toughest school to get into. “None of the places I have worked at in the last 13 years since I graduated high school have come close to mirroring the racial and socioeconomic diversity I grew up thinking was the norm in all of America,” she says. “As such, it’s never been difficult for me to see the power of privilege and the persistence of systemic racism at every stage of the STEM pipeline as I progressed through it.”
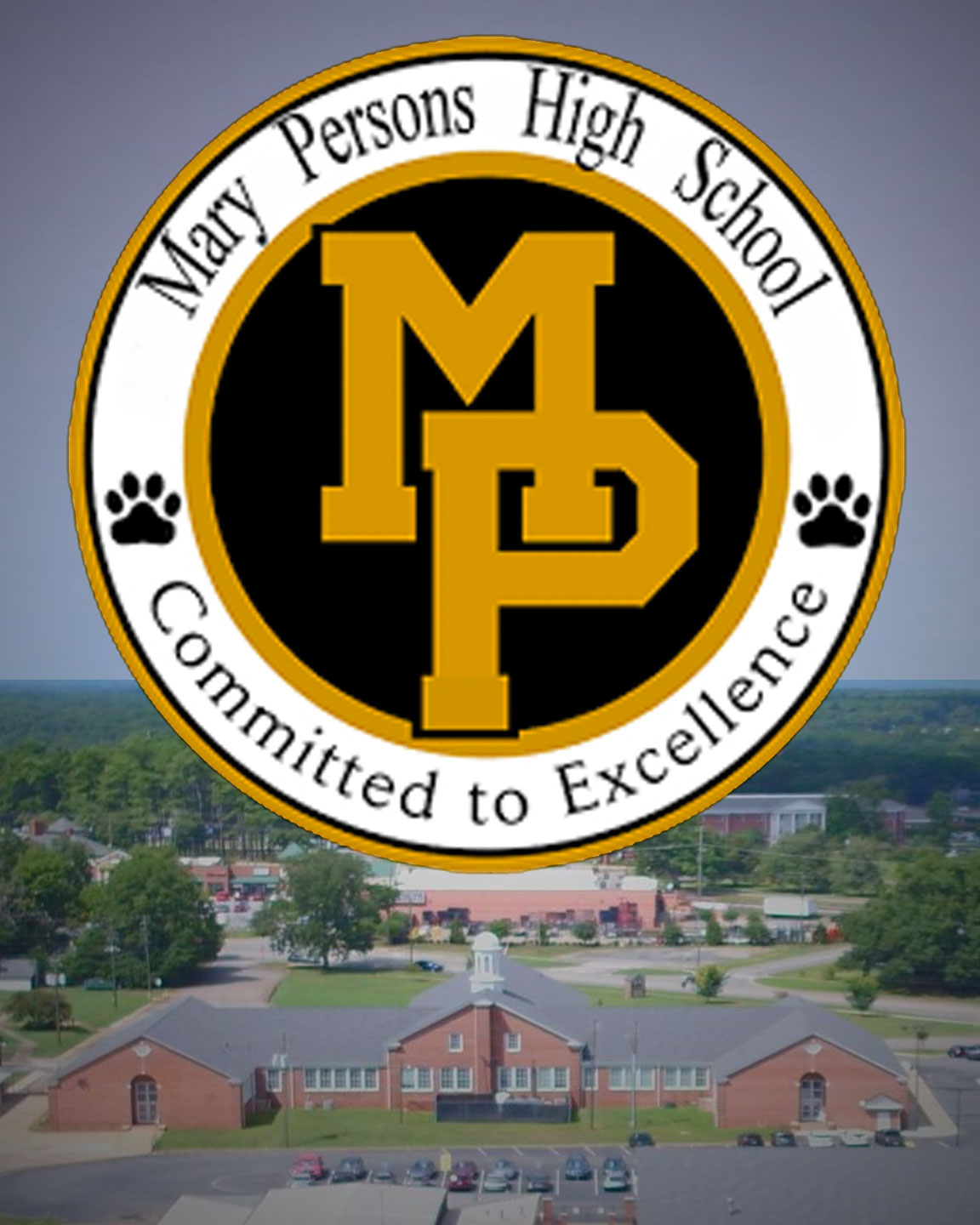
Mary Persons High School
Now an assistant professor in the Department of Atmospheric Sciences at the University of Utah, Haskins is savvier about her own seemingly unlikely journey into higher education. More importantly, perhaps, she’s keenly aware of the challenges “first-gen” college students and other underrepresented populations still face, having to navigate hurdles referred to as the “hidden curriculum” of academia. The term refers to things a neophyte in the academic world should know to maximize their experience and success but doesn’t. These are things that more privileged students tacitly understand or have been made aware of, like the norm of emailing potential professors to work with in graduate school before they submit their graduate applications or cluing into the notion that graduate students in STEM fields are often actually paid to go to school and do so without accruing debt from tuition.
Paying it Forward
Haskins’ unique perspective of these issues inspired her to use her second government stimulus check during the pandemic to fund a modest scholarship for an underrepresented minority student interested in pursuing an undergraduate STEM degree from her high school. This year, the scholarship went to Maleisha Jackson who is studying computer and robotics engineering at Kennesaw State University, located in north Georgia. “I think people really underestimate the impact that even receiving a 1,000 dollars can do for a student who needs it. I don’t know how I would have afforded a laptop and school supplies for my first year at MIT if I hadn’t received local scholarships like this one, and I want to pay that forward,” Haskins says.
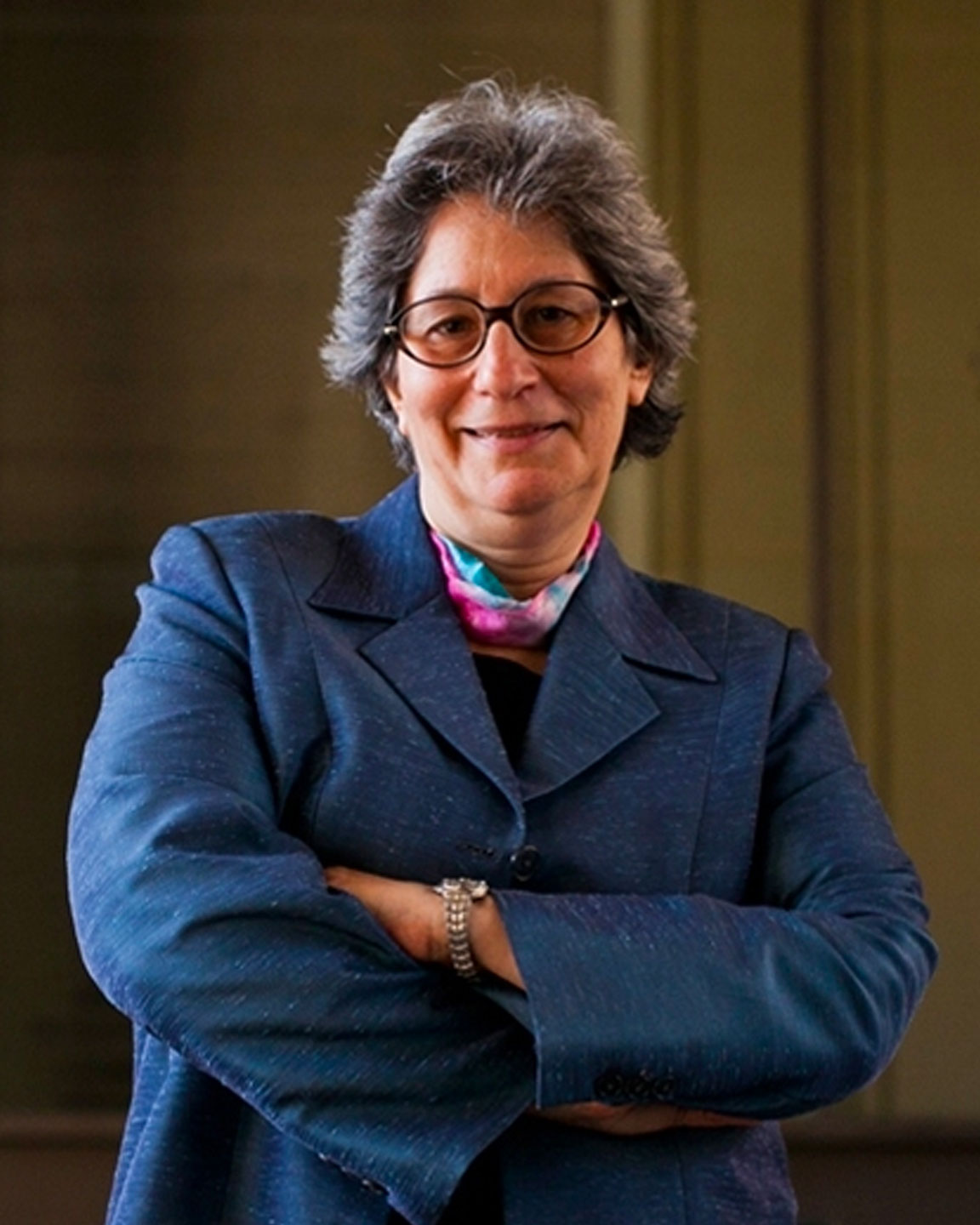
Professor Susan Solomon
Fortunately, MIT treated Haskins well, brokering an “externship” with NASA‘s Goddard Space Flight Center and providing an opportunity to work with Professor Susan Solomon, a 2007 Nobel Peace Prize co-recipient and a National Medal of Science winner awarded by the President. Solomon is best known for being the first to propose the chemical mechanism that is the cause of the Antarctic ozone hole. In the Solomon lab, the budding atmospheric scientist used MLS satellite data & balloon observations to explain fundamental chemical and meteorological differences that prohibit Arctic ozone loss from becoming as severe as Antarctic ozone loss, ultimately resulting in the publication of Haskins’ undergraduate research in the high impact journal, PNAS.
But even with the scholarship to MIT, Haskins required four years of Federal Pell grants and multiple campus jobs to make ends meet and says that even covering graduate application fees was difficult for her. When she was accepted to the University of Washington for graduate school, she was lucky enough to receive an ARCS Foundation fellowship she used to get herself cross-country to Seattle.
Compelling Challenges
Furnished with a PhD, she returned to MIT for a short stint as an NSF Postdoctoral Fellow before being hired by the U. Needless to say, it wasn’t for the theater that she and her wife moved to Utah’s capital city, but rather the unique (and to her, compelling) challenges facing the state, particularly the winter PM2.5 and summer ozone air quality issues impacting the Wasatch Front, especially during periodic weather “inversions” that trap emissions along the metropolitan valley. An expert in the chemistry of how chloride present in salt impacts air quality, particularly in the winter, Haskins noted, “there is no place in the United States that my research on air quality is more relevant to science and policy than it is in Salt Lake City."
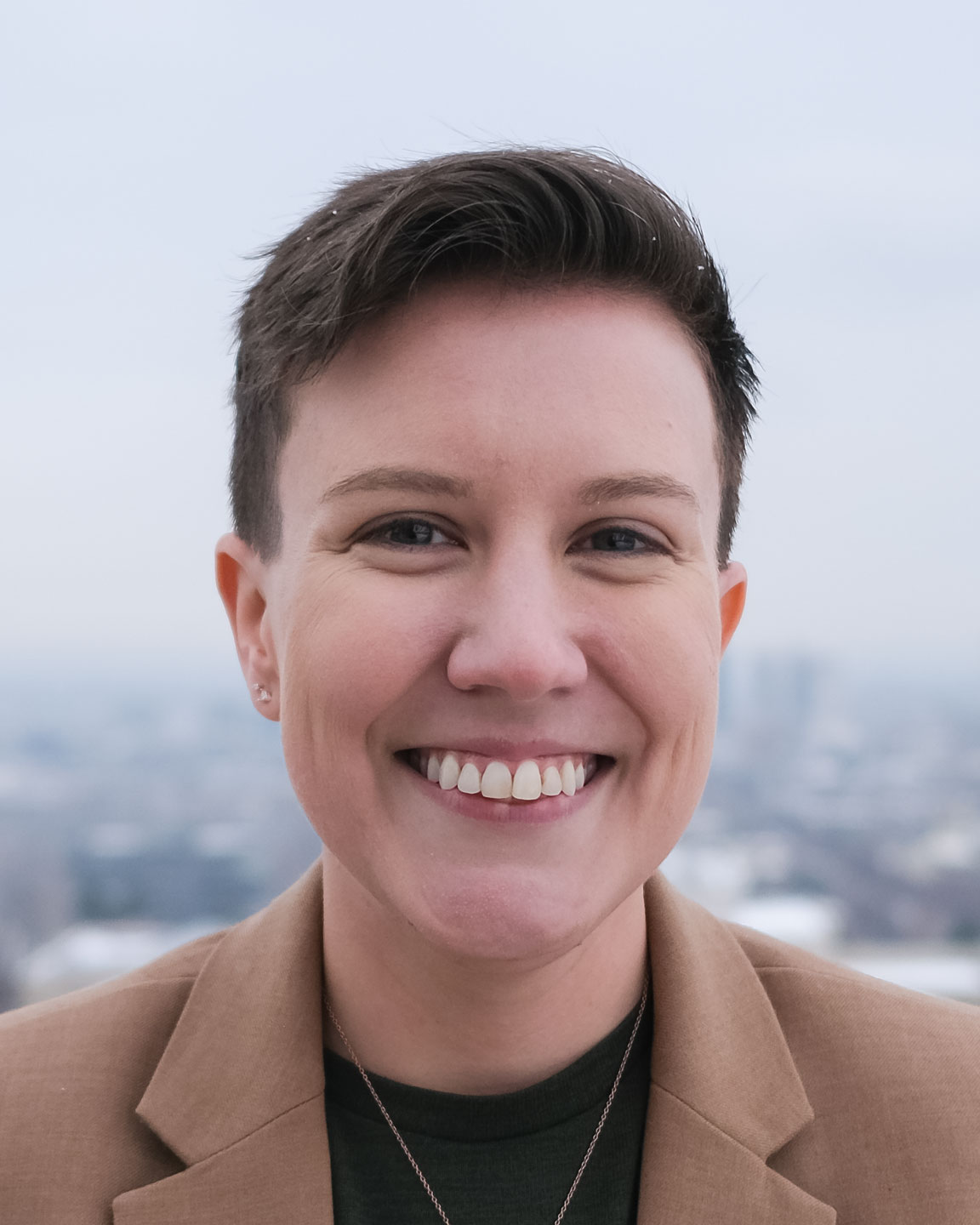
Jessica Haskins
Haskins’ research group at the U is focused on understanding and accurately modeling heterogeneous and multiphase chemistry that transforms natural and anthropogenic (human-derived) gas phase emissions into aerosol particles. These particles make up a key component of smog known as particulate matter (PM2.5). It turns out that, globally, exposure to PM2.5 is the fifth greatest risk factor for death, ranking only behind tobacco use and several other factors related to obesity. But in addition to their impact on human health, these aerosols formed through chemical reactions in the atmosphere also have direct impacts on climate and the Earth’s temperature by reflecting and absorbing light.
Today, more episodes of unhealthy air quality in the U.S., including in Salt Lake City, are experienced in the winter rather than summer, pointing to a shift in the chemistry responsible for formation of secondary pollutants like PM2.5, and ozone. This chemical regime shift has the unintended consequence of rendering past policy solutions to summer air quality issues largely ineffective in the winter. The ineffectiveness left scientists and policy makers with questions about how well they understand the underlying chemistry and what the most effective means are to mitigate such issues now and in a changing world. Haskins’ past and future research focuses on understanding this type of chemical shift through the lens of atmospheric chemistry with an eye towards understanding how future policy and climate solutions will impact the Earth’s temperature and air pollution formation.
Global Implications
The relevance of such research is not restricted to the intermountain west but has global implications. Large-population countries, like India and China, may have fewer interventions to maintain quality air such as EPA-recommended “scrubbers” on power plants, less stringent policies around automobile emissions and higher rates of open-air waste incineration. “I think what’s most exciting about the prospect of being here at the U,” says Haskins, “is the fact that what we learn about the drivers of variability in air pollution formation and how to control them here in Utah have a global relevance that can help inform policy makers in the East on the fastest and most effective ways to clean up their air quality.”
Haskins' interdisciplinary research sits at the intersection of atmospheric science and chemistry and strives to deepen our understanding of the complex cascade of reactions between our emissions and atmospheric oxidants. Those oxidants control how long gases like methane stay in the atmosphere. It’s a gumbo of considerations that turns, for Haskins, on her understanding of concentrations of common atmospheric oxidants like OH, O3, NO3, and Cl radicals that are dependent on everything from atmospheric water vapor concentrations, exposure to sunlight, temperature, aerosol surface area, emissions of gases like NOx from combustion, etc. She notes that “these processes are challenging to measure and therefore challenging to represent in models, and much remains to be discovered!”
Perhaps unique to her approach is the determination to centralize, assimilate and “exploit” the data already collected from satellites, observation networks, aircraft campaigns, government records and relevant available datasets to improve models. “One of the largest looming challenges our field faces now and, in the future, will be connecting an ever-growing dataset of highly localized measurements to scientifically accurate, but computationally efficient representations in predictive global models,” Haskins has written.
A Lot of Data
All of those data sets along with new ones yet to be collected are key to improving the accuracy and speed of global models of atmospheric composition. “Drawing on my experience in both the measurement and modeling community, my research program will serve to bridge this already significant but growing gap between the data we have and the data we use to inform predictive models and decision makers. Basically, we have a lot of data, and I want to use it,” Haskins says.
The upcoming projects in her group include re-analyzing old measurements to extract new constraints for models, new applications of machine learning and artificial intelligence to atmospheric chemistry problems and integrating data from product databases, patent applications, and other public records. “We’re still catching up with being able to efficiently use data from a variety of sources beyond just measurements made by those of us in academia–especially when you consider how rapidly new computation methods like machine learning have evolved,” she states. The application of artificial intelligence methods has only just begun to be applied to atmospheric chemistry problems, she explains, “but could greatly improve the speed and accuracy of our models.”
It's an exciting time to be an atmospheric scientist rooted in chemistry, and Jessica Haskins is looking forward to better understanding and communicating the relevant chemical drivers of variability in air pollution formation. But here in the high desert climate that has precious little in common with her Georgian home–except for that community theater thing–she is enthusiastic about building a diverse and collaborative research group in the Department of Atmospheric Sciences at the U and looks forward to preparing others for an auspicious career in science.