These good reasons are not mutually exclusive, and my own ideal, rarely achieved, is to combine all three. In case you are curious, the dull talk exemplified one of the bad reasons (it is hard), that I'll say no more about.
So what is this vaunted mathematical beauty? Is mathematical beauty the same as beauty in the arts and nature, or does it just happen to go by the same name?
Faced with a problem of this magnitude, poet and Distinguished Professor Katharine Coles and I decided to do what we do best. Talk about it. This year's Symposium on Science and Literature takes on the idea of beauty, bringing together poet Claudia Rankine, physicist Brian Greene, and neuroscientist/artist Bevil Conway for three days of discussion. As part of the preparation, we are jointly teaching a course this semester on the theme of Beauty to a small class of remarkable students, half from math, half from English. The English students are facing the trauma of making sense of math and physics and attempting to see the beauty therein. The Math students are facing the terror of making sense of complex poetry and attempting to see its beauty. And we are all taking on the collective challenge of reading philosophy to peek behind the curtain to ask what beauty is.
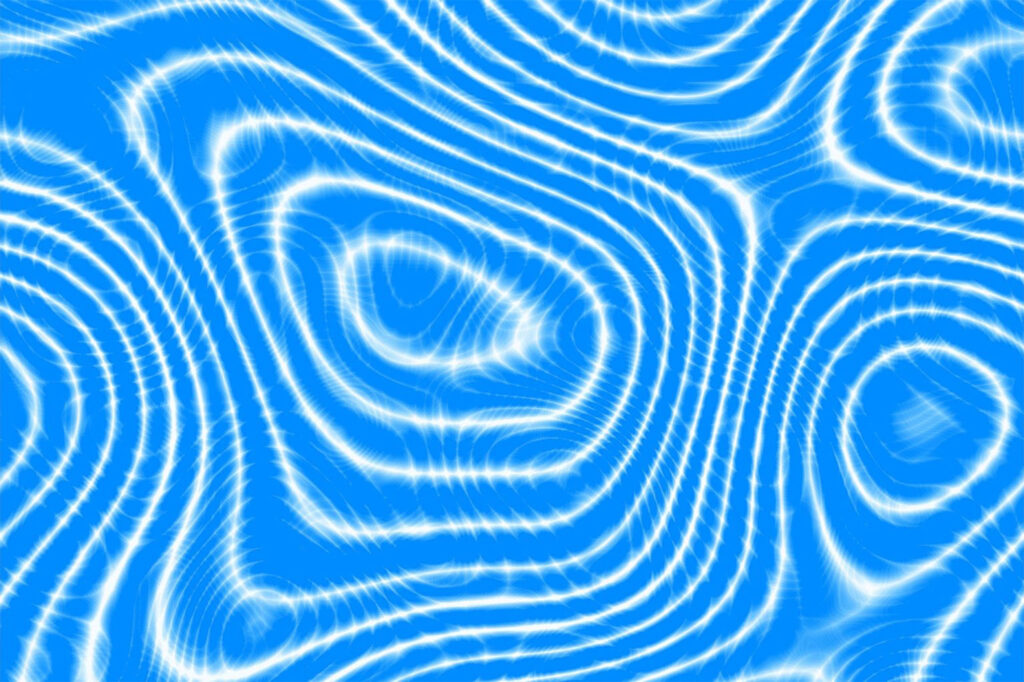
At the atomic scale, when one sheet of atoms arranged in a lattice is slightly offset from another sheet, moiré patterns can create some exciting and important physics with interesting and unusual electronic properties. (Image courtesy of Ken Golden)
Before revealing the answer, I'll share some of the mathematical ideas we have discussed, largely following the charming “The Joy of x” by Stephen Strogatz, inspired by his popular series for the New York Times online called "The Elements of Math.” Given the mixed group, the mathematics, in the spirit of Strogatz's book, is fundamental and not technical.
We began with an age-old question: What does the golden ratio have to do with rabbits? The golden ratio appears in geometry, describing the shape of a rectangle that is supposedly the most appealing to the eye, and appearing in the elegant logarithmic spiral. But this number also shows up as the limit of the ratio of the consecutive values of the Fibonacci sequence (1,1,2,3,5,8,13,21...). Each number is the sum of the previous two numbers, and the sequence can be generated by counting the population of immortal and fecund rabbits who produce babies every month and take just two months to mature. The beauty, we decided, lies in the unexpected connection of geometry and arithmetic.
The most elegant and venerable link between geometry and numbers is the Pythagorean theorem, that the sum of the squares of the sides of right triangle is equal to the square of the hypotenuse. Where do those squares come from anyway? I know three broad classes of proof. The first is rather pretty, involving drawing squares on the sides and hypotenuse and cleverly chopping them to get them to match. The second, which I came up with when I couldn't figure out how to do the first, is rather ugly, involving drawing lines, taking ratios, and doing a bunch of nasty algebra. The best proof, which I had not seen before, was attributed to the teenage Einstein in one of the books we read for the class ``A Beautiful Question" by Nobel-prize winning physicist Frank Wilczek. It is based on what we mean by area. If you take any shape and make it twice as big by stretching equally in all directions, the area gets bigger by a factor of 4. That's where the squares come from — if you made the shape 3 times as big, the area would be 3^2=9 times bigger. Rather than building on tricky drawing or algebra, this proof requires adding just one line to the picture, and then thinking. In mathematics, beauty lies in deep simplicity. And, as in music and the arts, that kind of simplicity has to be earned.
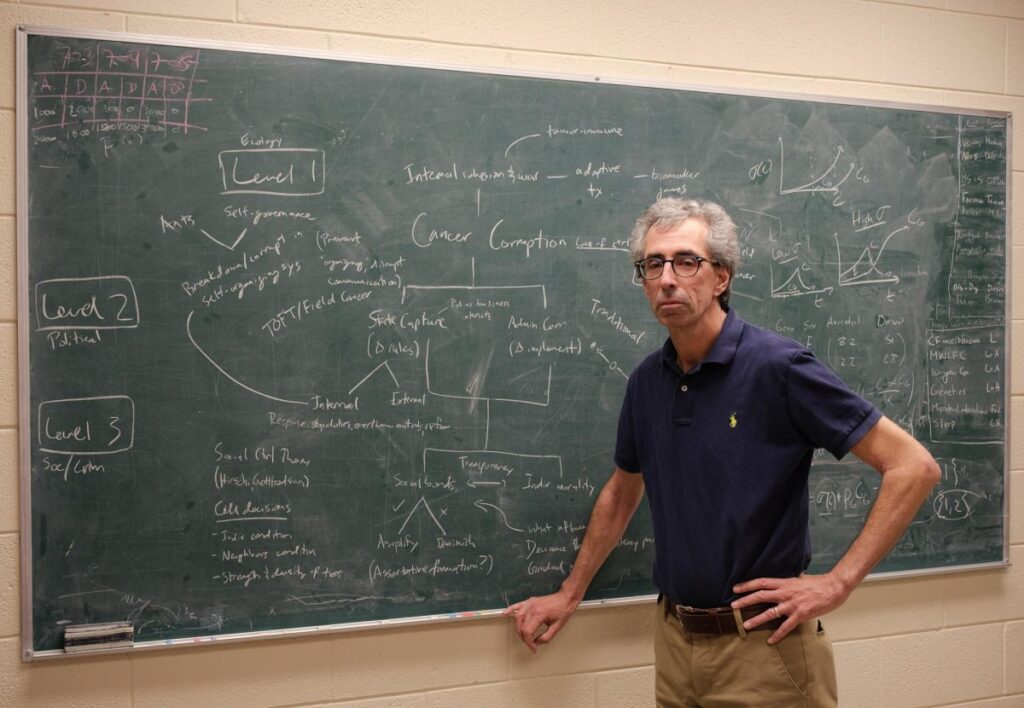
Fred Adler writes equations inside his office at the University of Utah in Salt Lake City on Sept. 5, 2023. (Photo by Marco Lozzi | The Daily Utah Chronicle)
I became interested in mathematics because of the magic of numbers. And large numbers have an allure all their own. The Fibonacci series, like rabbit populations, grows rather fast. But what if you want to write down really huge numbers? We can use the way that mathematical ideas build on themselves, recalling the progression of arithmetic in elementary school. Addition is repeated counting (6+7=13 means counting to six and then counting to seven). Multiplication is repeated addition (6*7=42 means adding up seven 6's). Exponentiation is repeated multiplication (6^7=279936 means 6*6*6*6*6*6*6, multiplying together seven 6's). The numbers are starting to get pretty big. But to really turbocharge, let's try repeated exponentiation. Donald Knuth invented "arrow notation" to handle this question. 6↑↑7 is 6 raised to the 6th power seven times, or 6^6^6^6^6^6^6. There's really no way to say how big this number is. Even 6↑↑3 has 36,305 digits written in decimal notation. But no matter how absurdly large these numbers become, they are still nothing compared with infinity. The beautiful has the sense of the inexhaustible, the beauty of a poem, the face of one you love.
We have touched on many other mathematical questions. Is the quadratic formula ugly, or does it have "inner beauty"? Is there a beautiful poetry behind the existential angst of probabilities? Will I ever get over my prejudice against fractals?
Along the way, we've learned a few things. Good things happen when geometry and algebra get together. Beauty has an element of surprise, evoked by connections between apparently different things. Beauty arises when complexity meets simplicity and when simplicity meets complexity. Einstein was a beautiful and deep thinker. Keats was a great poet who evoked deep thoughts with beautiful words.
There is a toast attributed variously to G.H. Hardy and other famous mathematicians: “Here’s to pure mathematics. May it never be useful for anything!” The Enlightenment philosopher Immanuel Kant argues that beauty indeed must lie outside anything useful, attractive or even morally good. But mathematics has the remarkable power to surprise us with beauty when it seeks to be useful, and with usefulness when it seeks beauty.
Fred Adler is Professor of Mathematics and Director of the School of Biological Sciences at the University of Utah.
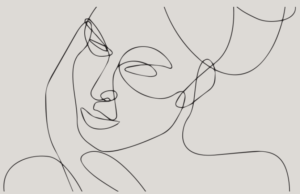
The 2024 Science and Literature Symposium takes place April 10-12. This year's topic arises from reexaminations of beauty that are occurring broadly not only in the arts and across such disciplines as ethnic and disability studies, but also in biology, where dominant theories about the possible evolutionary purposes of beauty are being questioned.