Is our home galaxy, the Milky Way Galaxy, a special place? A team of scientists started a journey to answer this question more than a decade ago. Commenced in 2013, the Satellites Around Galactic Analogs (SAGA) Survey studies galaxy systems like the Milky Way. Now, the SAGA Survey just published three new research articles that provide us with new insights into the uniqueness of our own Milky Way Galaxy after completing the census of 101 satellite systems similar to the Milky Way’s.
These “satellites” are smaller galaxies in both mass and size which orbit a larger galaxy, usually called the host galaxy. Just as with smaller satellites that orbit the Earth, these satellite galaxies are captured by the gravitational pull of the massive host galaxy and its surrounding dark matter. The Milky Way Galaxy is the host galaxy of several satellite galaxies, of which the two largest are the Large and Small Magellanic Clouds (LMC and SMC). While LMC and SMC are visible to the naked eye from the Southern Hemisphere, there are many other fainter satellite galaxies orbiting around the Milky Way Galaxy that can only be observed with a large telescope.
The goal of the SAGA Survey is to characterize satellite systems around other host galaxies that have similar stellar masses as the Milky Way Galaxy. Yao-Yuan Mao, a University of Utah faculty member in the Department of Physics and Astronomy, is co-leading the SAGA Survey with Marla Geha at Yale University and Risa Wechsler at Stanford University. Mao is the lead author of the first article in the series of three that have all been accepted by the Astrophysical Journal. This series of articles reports on the SAGA Survey’s latest findings and makes the survey data available to other researchers worldwide.
An outlier galaxy?
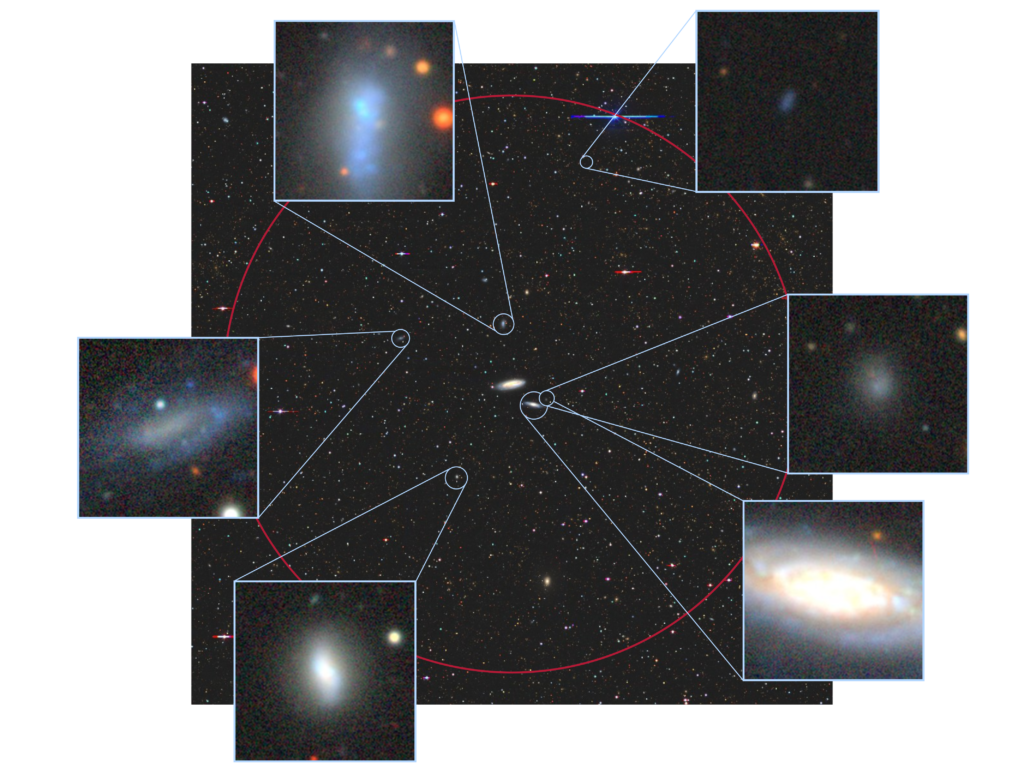
An image of a Milky Way-like galaxy and its system of satellite galaxies. The SAGA survey identified six small satellite galaxies in orbit around this Milky Way analog. Credit: Yasmeen Asali (Yale), with images from the DESI Legacy Surveys Sky Viewer https://www.legacysurvey.org/acknowledgment/
In the first study led by Mao, the researchers highlighted 378 satellite galaxies identified across 101 Milky Way-mass systems. The number of confirmed satellites per system ranged from zero to 13 — compared to four satellites for the Milky Way. While the number of satellite galaxies in the Milky Way system is on par with the other Milky Way-mass systems, “the Milky Way appears to host fewer satellites if you consider the existence of the LMC,” Mao said. The SAGA Survey has found that systems with a massive satellite like the LMC tend to have a higher total number of satellites, and our Milky Way seems to be an outlier in this regard.
An explanation for this apparent difference between the Milky Way and the SAGA systems is the fact that the Milky Way has only acquired the LMC and SMC quite recently, compared with the age of the universe). The SAGA article explains that if the Milky Way Galaxy is an older, slightly less massive host with the recently added LMC and SMC, one would then expect a lower number of satellites in the Milky Way system not counting other smaller satellites that LMC/SMC might have brought in.
This result demonstrates the importance of understanding the interaction between the host galaxy and the satellite galaxies, especially when interpreting what we learn from observing the Milky Way. Ekta Patel, a NASA Hubble Postdoctoral Fellow at the U but not part of the SAGA team, studies the orbital histories of Milky Way satellites. After learning about the SAGA results, Patel said, “Though we cannot yet study the orbital histories of satellites around SAGA hosts, the latest SAGA data release includes a factor of ten more Milky Way-like systems that host an LMC-like companion than previously known. This huge advancement provides more than 30 galaxy ecosystems to compare with our own, and will be especially useful in understanding the impact of a massive satellite analogous to the LMC on the systems they reside in.”
Why do galaxies stop forming stars?
The second SAGA study of the series is led by Geha, and it explores whether these satellite galaxies are still forming stars. Understanding the mechanisms that would stop the star formation in these small galaxies is an important question in the field
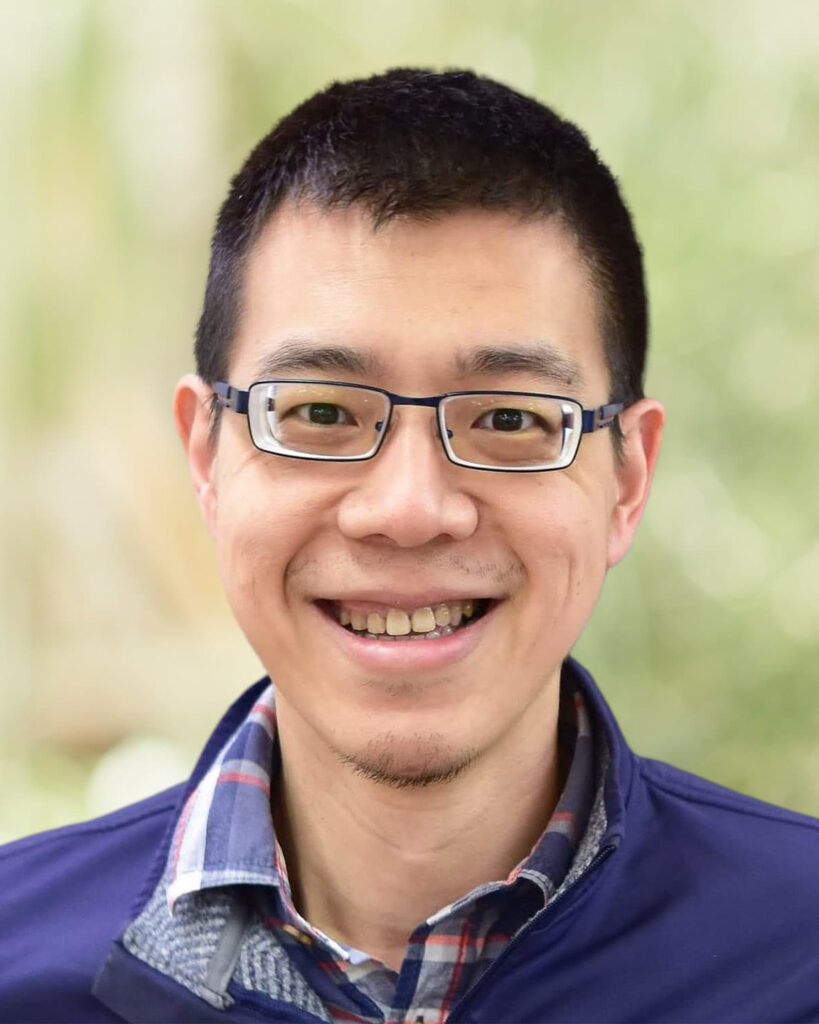
Yao-Yuan Mao
of galaxy evolution. The researchers found, for example, that satellite galaxies located closer to their host galaxy were more likely to have their star formation “quenched,” or suppressed. This suggests that environmental factors help shape the life cycle of small satellite galaxies.
The third new study is led by Yunchong (Richie) Wang, who obtained his PhD with Wechsler. This study uses the SAGA Survey results to improve existing theoretical models of galaxy formation. Based on the number of quenched satellites in these Milky Way-mass systems, this model predicts quenched galaxies should also exist in more isolated environments — a prediction that should be possible to test in the coming years with other astronomical surveys such as the Dark Energy Spectroscopic Instrument Survey.
Gift to the astronomy community
In addition to these exciting results that will enhance our understanding of galaxy evolution, the SAGA Survey team also brings a gift to the astronomy community. As part of this series of studies, the SAGA Survey team published new distance measurements, or redshifts, for about 46,000 galaxies. “Finding these satellite galaxies is like finding needles in a haystack. We had to measure the redshifts for hundreds of galaxies to just identify one satellite galaxy,” Mao said. “These new galaxy redshifts will enable the astronomy community to study a wide range of topics beyond the satellite galaxies.”
The SAGA Survey was supported in part by the National Science Foundation and the Heising-Simons Foundation. Other authors of these three SAGA studies include Yasmeen Asali, Erin Kado-Fong, Nitya Kallivayalil, Ethan Nadler, Erik Tollerud, Benjamin Weiner, Mia de los Reyes, John F. Wu, Tom Abel, and Peter Behroozi.