What do cycling and rocks have to do with each other?
July 15, 2024
University of Utah geologists Peter Lippert and Sean Hutchings are helping bring attention to the hidden star of a major sporting event this summer.
I’m not talking about the Olympics, but the Tour de France, which kicked off on June 29 in Florence, Italy and will finish July 21 in Nice, France. This is the first time the iconic bicycle race won’t finish in Paris, due to the city hosting the Summer Olympics.
The star they’re highlighting rises above the competition, literally. It’s also below and all around.
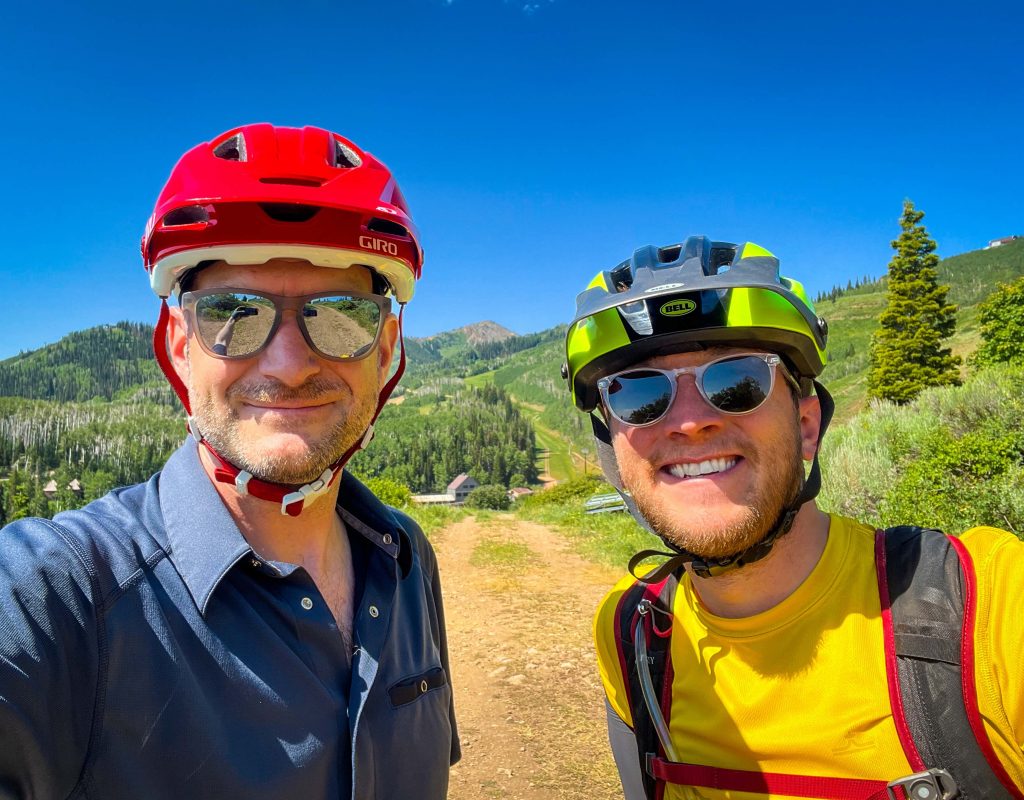
Peter Lippert and Sean Hutchings
The Geo Tour de France project (Geo TdF) is a blog exploring the geology of the various stages of the bike race. Lippert and Hutchings are two of the five North American contributors to the blog this year. They covered Stage 14, a 152-kilometer ride through the Pyrenees held Saturday and won Saturday by overall race leader Tadej Pogacar of Slovenia in just over four hours.
“The centerpiece of the stage is the Col du Tourmalet, a very famous fabled climb in the Tour de France that has lots of amazing history,” said Lippert, an associate professor in the Department of Geology & Geophysics and director of the Utah Paleomagnetic Center. “This is going to be one of the really decisive stages of the Tour this year.”
The entire race covers 3,500 kilometers (2,175 miles) in 21 stages.
“I’ve always loved this project, because it’s just such a fun way to share our science and share how we see the world with the public and particularly a public that’s probably not often thinking about the geology,” Lippert said.
For Lippert and Hutchings, as well as many of their peers across the world, geology and cycling go hand in hand.
“Riding a bike up and down a mountain gives you a lot of time to see how the mountains put together the rocks you’re riding over in the landscapes that you’re on,” Lippert said. “We’re both trained geologists for most of our lives so it’s hard not to always be thinking about [geology].”
Utah in particular boasts captivating and diverse geological features.
“It’s mountain biking Candyland around here,” said Hutchings, a graduate research assistant in the U of U Seismograph Stations. “It’s fun to be able to climb up to the top of the hill and it’s hard to not interact with rocks on the way as well.”
“You have this new identity with the landscape you’re on if you’re able to understand what’s going on beneath your feet and what made the landscape,” Lippert said. “I think cycling is a really great high impact sense of place type of experience. You’re going a little bit slower. You get to look around.”
Geo Tour de France project
This same sentiment was the original inspiration for Geo TdF project creator Douwe van Hinsbergen, professor of geology at the Netherlands’ Utrecht University.
“He wanted to explore a different way of sharing geology with the public,” Lippert said. “This is a total goldmine.’
Fans who watch the livestream of the race are inadvertently watching hours of spectacular geological features. The Geo TdF project enhances the viewing experience by telling geological stories that ground the competition in the larger history of the landscape.
Lippert first contributed to the blog two years ago, and this time around included Hutchings. The pair worked together during Hutchings’ bachelor’s degree at the U and often bike together.
“I know nothing about Pyrenean geology, so this was a great learning opportunity for me,” Hutchings said. “For graduate school, I’ve dipped more into the seismology realm, so getting back to my geology roots was a fun exercise.”
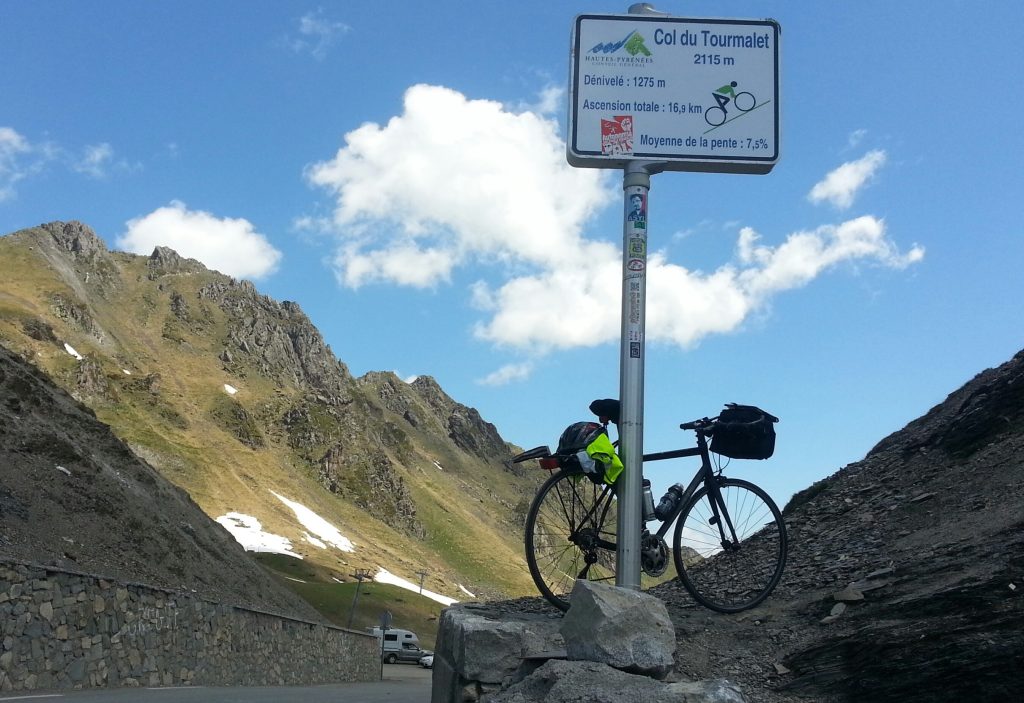
Col du Tourmalet. Photo credit: Gilles Guillamot, Wikimedia Commons
Tectonic training camp
Stage 14 passed through Pyrenees, the mountains on France’s border with Spain, with an average grade of 7.9%. That’s just under 95 miles at an average grade more than twice as steep as the incline from President’s Circle to the Natural History Museum of Utah.
“Let’s think big” is what Lippert and Hutchings thought when they were presented with the opportunity to cover this pivotal stage of the race.
“I mean the Tour de France is big, the Pyrenees are big, tectonics are big. Sean is more of a geophysicist working with earthquakes and things like that,” Lippert said. “My expertise is in collisional mountain builds, like what happens when oceans close and mountains form. So we thought let’s just go back to basics and keep it big.”
What could be bigger than beginning with the ancient supercontinent Pangea? For their portion of the project, Lippert and Hutchings focused on the creation of the Pyrenees mountain range which began with the separation of Pangea and subsequent plate collisions, a process they describe as a “tectonic training camp.”
A Wealth of information
Some readers might be wondering if these passionate geologists will eventually run out of topics to discuss, even though the Tour course changes each year. Lippert and Hutchings aren’t concerned about that at all.
“One nice thing about geology is that rocks usually stay put and you can go back to check them out year after year. So the rocks don’t change, but the way that we can talk about them does. The limit is our creativity now, what the rocks can provide, because they’re full of really good stories,” Lippert said. “There’s a wealth of information that a single rock can tell you. Where it came from, and the time it took to get there, and what it looked like at the time.”
By Lauren Wigod