National Academy of Sciences
Valeria Molinero elected as a member of the National Academy of Sciences.
Molinero is the Jack and Peg Simons Endowed Professor of Theoretical Chemistry and the director of the Henry Eyring Center for Theoretical Chemistry. She is a theoretical chemist and uses computer and statistical models to explore the science of how crystals form and how matter changes from one phase to another down to the atomic scale.
Much of her work has involved the transition between water and ice and how that transition occurs in the formation of clouds, in insects with antifreeze proteins, and in food products, especially those containing sugars. Her work has implications for any process in which control of the formation and growth of ice crystals is critical, including snowmaking at ski resorts, protection of crops from freezing, preservation of human organs and tissue for transplant, and production of ice cream and gelato, her favorite food. In 2020, she and her international colleagues demonstrated that the smallest possible nanodroplet of water that can freeze into ice is around 90 molecules, a finding that earned them the 2020 Cozzarelli Prize from the journal Proceedings of the National Academy of Sciences.
She is a fellow of the American Academy of Arts & Sciences and recipient of several U awards, including the Distinguished Scholarly and Creative Research Award in 2019, the Extraordinary Faculty Achievement Award in 2016, the Camille Dreyfus Teacher-Scholar Award in 2012 and the College of Science Myriad Faculty Award for Research Excellence in 2011. She has also been honored by the Beckman Foundation with its Young Investigator Award, and by the International Association for the Properties of Water and Steam with its Helmholtz Award.
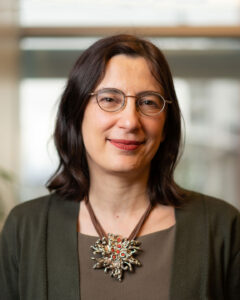
Valeria Molina
"There’s satisfaction that comes from seeing someone grow from the beginning of the Ph.D. into an accomplished researcher."
Valeria heard about her election between the news of a new publication with postdoctoral scholar Debdas Dhabal and preparations for a doctoral student’s dissertation defense. She received a phone call from colleague Dale Poulter, a distinguished professor emeritus and National Academy of Sciences member, to announce her election. “I was shocked,” she says. “To say it was a surprise would not do it justice. It was fantastic.”
Minutes later, she went into the dissertation defense, reflecting on the range of accomplishments represented by the publication, the election and the defense. “All the research is made essentially there, in the work of the students and postdocs,” she says. “There’s satisfaction that comes from seeing someone grow from the beginning of the Ph.D. into an accomplished researcher.”
Molinero is among 120 U.S. scientist-scholars and 30 foreign associates elected at the Academy’s Annual Meeting in Washington, D.C. She joins 16 other current University of Utah researchers who’ve been elected to the Academy. The National Academies, which also include the National Academy of Engineering and National Academy of Medicine, recognizes scholars and researchers for significant achievements in their fields and advise the federal government and other organizations about science, engineering and health policy. With today’s elections, the number of National Academy of Sciences members stands at 2,512, with 517 foreign associates.
Read more at nasaonline.org.
Past & Present
- National Academy of Sciences:
Brenda Bass, Cynthia Burrows, Mario Capecchi, Dana Carroll, Thure Cerling, James Ehleringer, Kristen Hawkes, James O’Connell, Baldomero “Toto” Olivera, C. Dale Poulter, Peter Stang, Wesley Sundquist, Polly Wiessner, Henry Harpending, Jesse D. Jennings, Erik Jorgensen, Cheves Walling, Sidney Velick, John R. Roth, Josef Michl, Ray White, Julian Steward, Jeremy Sabloff, Henry Eyring and Louis Goodman and Mary C. Beckerle. - National Academy of Engineering:
Jindrich Kopecek, R. Peter King, Adel Sarofim, Sung Wan Kim, Gerald Stringfellow, Donald Dahlstrom, George Hill, Jan D. Miller, Milton E. Wadsworth, Thomas G. Stockham, John Herbst, Stephen C. Jacobsen, Willem J. Kolff, Alex G. Oblad, Anil Virkar and William A. Hustrulid. - National Academy of Medicine:
Mario Capecchi, Wendy Chapman, Sung Wan Kim, Vivian Lee, Baldomero “Toto” Olivera, Stephen C. Jacobsen, Eli Adashi, Paul D. Clayton and Homer R. Warner.