After months of earth-moving, the Applied Science Project gets some serious hardware.
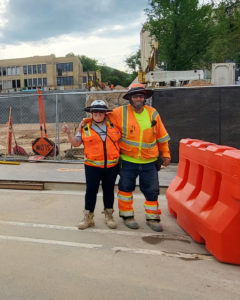
Senior Risk and Safety Manager Carlyn Chester and flagman Alan. Credit: David Pace
These days pedestrians along University Street on the westside of campus are typically met by Alan, a bearded, sixty-something employee and certified UDOT flagger dressed in Okland Construction garb, including hard hat with neck sunshade that cascades to his shoulders and sometimes flaps in the wind. Alan’s here keeping order at the gated threshold to the construction site of the Applied Science Project and is happy to give you a fist bump as you walk to work along the detour they’ve put up. Armed with a push broom, and a mobile phone, he slows traffic for entering and exiting trucks, and lines up arrivals carrying everything from timbers to a porta-potty called “Honey Bucket.”
On the morning of June 7th, the team was preparing for the delivery of a giant tower crane, in sections, which will stand around 265 feet tall for a full year at the site like a giant Tinker Toy. A tower crane features a jib or “jib arm” as a horizontal beam used to support the load clear of the main support. It can typically lift 19.8 tons (18 metric tons).
“So these cranes are so big they need to have all these counterweights and stuff,” says Carlyn Chester BS’09, Senior Risk and Safety Manager at Okland Construction. She spells her name for us: “Like George Carlin [the late sometimes raunchy comedian] but with a ‘y’ . . . and I’m not a dirty old man,” she says with a laugh. Chester oversees all of the many Okland projects at the University of Utah. The cranes need to be “strong enough to pick up those tower pieces,” she continues over the relentless beeping of a nearby steer loader pushing gravel. “You need a crane to build a crane. You have to put in all the counterweights and footings . . . [There are] two big semis worth of materials just to get that crane set up tomorrow before the tower crane comes in.”
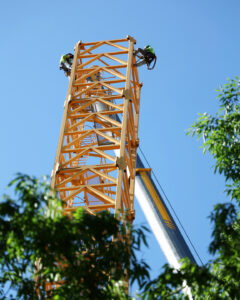
Credit: Todd Anderson
The gaping hole in front of the old Stewart Building—site of the new Applied Science Building—is squared off with wooden bulwarks holding up the sides (temporary) backed by a cement retaining wall (permanent). It looks like a neatly squared-off grave for a giant of sorts, two stories deep at the back and the sides sloping down the hill to a mere curb at the street. It’s a striking contrast to the bucolic Cottam’s Gulch with its brick path and towering hybrid trees to the north which will be a preserved historic asset to what will become the College of Science’s Crocker Science Complex.
“Did you find any bones?” we ask. Back in 2017 when the George Thomas Building was being retrofitted and expanded for the Crocker Science Center, Okland unearthed human bones that turned out to be the remains of old cadavers that had been discarded decades earlier by the medical school, originally located in the Life Sciences Building, another Okland project on campus.
“We found a couple of things,” says Chester. “The paleontologist people were here every single day when we were digging.” (It turns out the bones were from modern animals.)
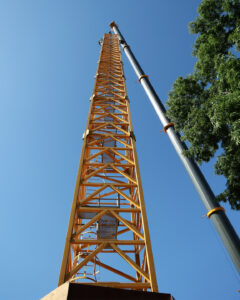
Credit: Todd Anderson
Carlyn points at a boxy, hexagonal structure to the left where a temporary footing has been positioned in the bottom of it. The footing is inspected by a structural engineer “to make sure it’s level and plumb so that when we start building, [the tower crane is] stable. There’s so much science that goes into it and mathematics,” she says.
Meanwhile, Alan has ambled back to the street to talk to a truck driver who has just pulled up. When he returns, he and Carlyn pose for a picture together–all smiles under their hard hats and neck shades that faintly remind one of Lawrence of Arabia’s. Alone, we ask Alan to “Flash the U” for us which he struggles a bit with. “My dad went to BYU,” he says sheepishly.
Bright and early next morning, Alan was back giving his signature fist bumps to passers-by. They stopped for a few moments to witness the newly arrived crane-to-build-a-crane with a synchronized telescoping boom as high (or higher) than one of those vertiginous, gut-wrenching rides at Lagoon amusement park north of here. Soon the semis arrived with tower segments which were off-loaded, rigged and then lofted off the ground vertically.
Even the rowdy fox squirrels in Cottam’s Gulch paused in a moment of awe as the Tinker Toy began to rise, a flash of yellow latticed steel against the summer sky.
By David Pace