HIV Microscopy
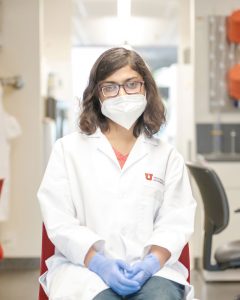
Ipsita Saha, graduate research assistant
Pioneering method reveals dynamic structure in HIV.
Viruses are scary. They invade our cells like invisible armies, and each type brings its own strategy of attack. While viruses devastate communities of humans and animals, scientists scramble to fight back. Many utilize electron microscopy, a tool that can “see” what individual molecules in the virus are doing. Yet even the most sophisticated technology requires that the sample be frozen and immobilized to get the highest resolution.
Now, physicists from the University of Utah have pioneered a way of imaging virus-like particles in real time, at room temperature, with impressive resolution. In a new study, the method reveals that the lattice, which forms the major structural component of the human immunodeficiency virus (HIV), is dynamic. The discovery of a diffusing lattice made from Gag and GagPol proteins, long considered to be completely static, opens up potential new therapies.
When HIV particles bud from an infected cell, the viruses experience a lag time before they become infectious. Protease, an enzyme that is embedded as a half-molecule in GagPol proteins, must bond to other similar molecules in a process called dimerization. This triggers the viral maturation that leads to infectious particles. No one knows how these half protease molecules find each other and dimerize, but it may have to do with the rearrangement of the lattice formed by Gag and GagPol proteins that lay just inside of the viral envelope. Gag is the major structural protein and has been shown to be enough to assemble virus-like particles. Gag molecules form a lattice hexagonal structure that intertwines with itself with miniscule gaps interspersed. The new method showed that the Gag protein lattice is not a static one.
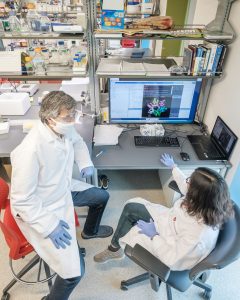
The Saffarian Lab in the Crocker Science Center
“This method is one step ahead by using microscopy that traditionally only gives static information. In addition to new microscopy methods, we used a mathematical model and biochemical experiments to verify the lattice dynamics,” said lead author Ipsita Saha, graduate research assistant at the U’s Department of Physics & Astronomy. “Apart from the virus, a major implication of the method is that you can see how molecules move around in a cell. You can study any biomedical structure with this.”
The paper published in Biophysical Journal on June 26, 2020.
Mapping a nanomachine.
The scientists weren’t looking for dynamic structures at first—they just wanted to study the Gag protein lattice. Saha led the two year effort to “hack” microscopy techniques to be able to study virus particles at room temperature to observe their behavior in real life. The scale of the virus is miniscule — about 120 nanometers in diameter—so Saha used interferometric photoactivated localization microscopy (iPALM).
First, Saha tagged the Gag with a fluorescent protein called Dendra2 and produced virus-like particles of the resulting Gag-Dendra2 proteins. These virus-like particles are the same as HIV particles, but made only of the Gag-Dendra2 protein lattice structure. Saha showed that the resulting Gag-Dendra2 proteins assembled the virus-like particles the same way as virus-like particle made up regular Gag proteins. The fluorescent attachment allowed iPALM to image the particle with a 10 nanometer resolution. The scientists found that each immobilized virus-like particle incorporated 1400 to 2400 Gag-Dendra2 proteins arranged in a hexagonal lattice. When they used the iPALM data to reconstruct a time-lapse image of the lattice, it appeared that the lattice of Gag-Dendra2 were not static over time. To make sure, they independently verified it in two ways: mathematically and biochemically.
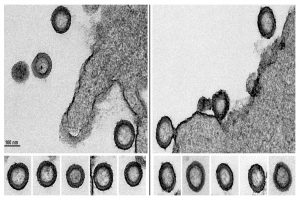
80 nm sections of cells (2020 Biphys Journal) - Saha & Saffarian
Initially, they divided up the protein lattice into uniform separate segments. Using a correlation analysis, they tested how each segment correlated with itself over time, from 10 to 100 seconds. If each segment continued to correlate with itself, the proteins were stationary. If they lost correlation, the proteins had diffused. They found that over time, the proteins were quite dynamic.
The second way they verified the dynamic lattice was biochemically. For this experiment, they created virus-like particles whose lattice consisted of 80% of Gag wild type proteins, 10% of Gag tagged with SNAP, and 10% of gag tagged with Halo. SNAP and Halo are proteins that can bind a linker which binds them together forever. The idea was to identify whether the molecules in the protein lattice stayed stationary, or if they migrated positions.
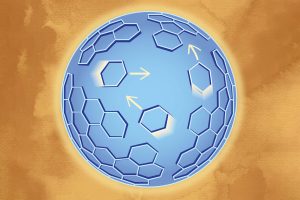
Rendering of Gag molecules proteins diffusing across a virus-like particle - Dave Meikle/Saffarian Lab
“The Gag-proteins assemble themselves randomly. The SNAP and Halo molecules could be anywhere within the lattice—some may be close to one another, and some will be far away,” Saha said. “If the lattice changes, there’s a chance that the molecules come close to one another.”
Saha introduced a molecule called Haxs8 into the virus-like particles. Haxs8 is a dimerizer—a molecule that covalently binds SNAP and Halo proteins when they are within binding radius of one another. If SNAP or Halo molecules move next to each other, they’ll produce a dimerized complex. She tracked these dimerized complex concentrations over time. If the concentration changed, it would indicate that new pairs of molecules found each other. If the concentration decreased, it would indicate the proteins broke apart. Either way, it would indicate that movement had taken place. They found that over time, the percentage of the dimerized complex increased; HALO and SNAP Gag proteins were moving all over the lattice and coming together over time.
A new tool to study viruses.
This is the first study to show that the protein lattice structure of an enveloped virus is dynamic. This new tool will be important to better understand the changes that occur within the lattice as new virus particles go from immaturity to dangerously infectious.
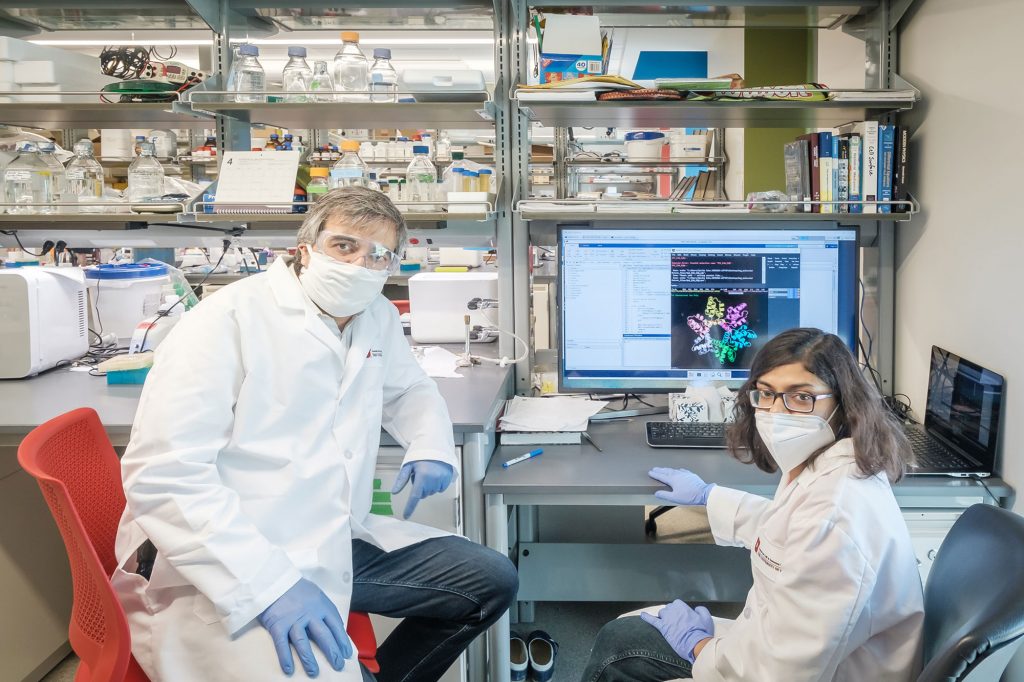
Saveez Saffarian and Ipsita Saha
“What are the molecular mechanisms that lead to infection? It opens up a new line of study,” said Saha. “If you can figure out that process, maybe you can do something to prevent them from finding each other, like a type of drug that would stop the virus in its tracks.”
Saveez Saffarian, professor in the Department of Physics & Astronomy at the U, was senior author on the paper.