bio-news
Ty Mellor
Ty Mellor
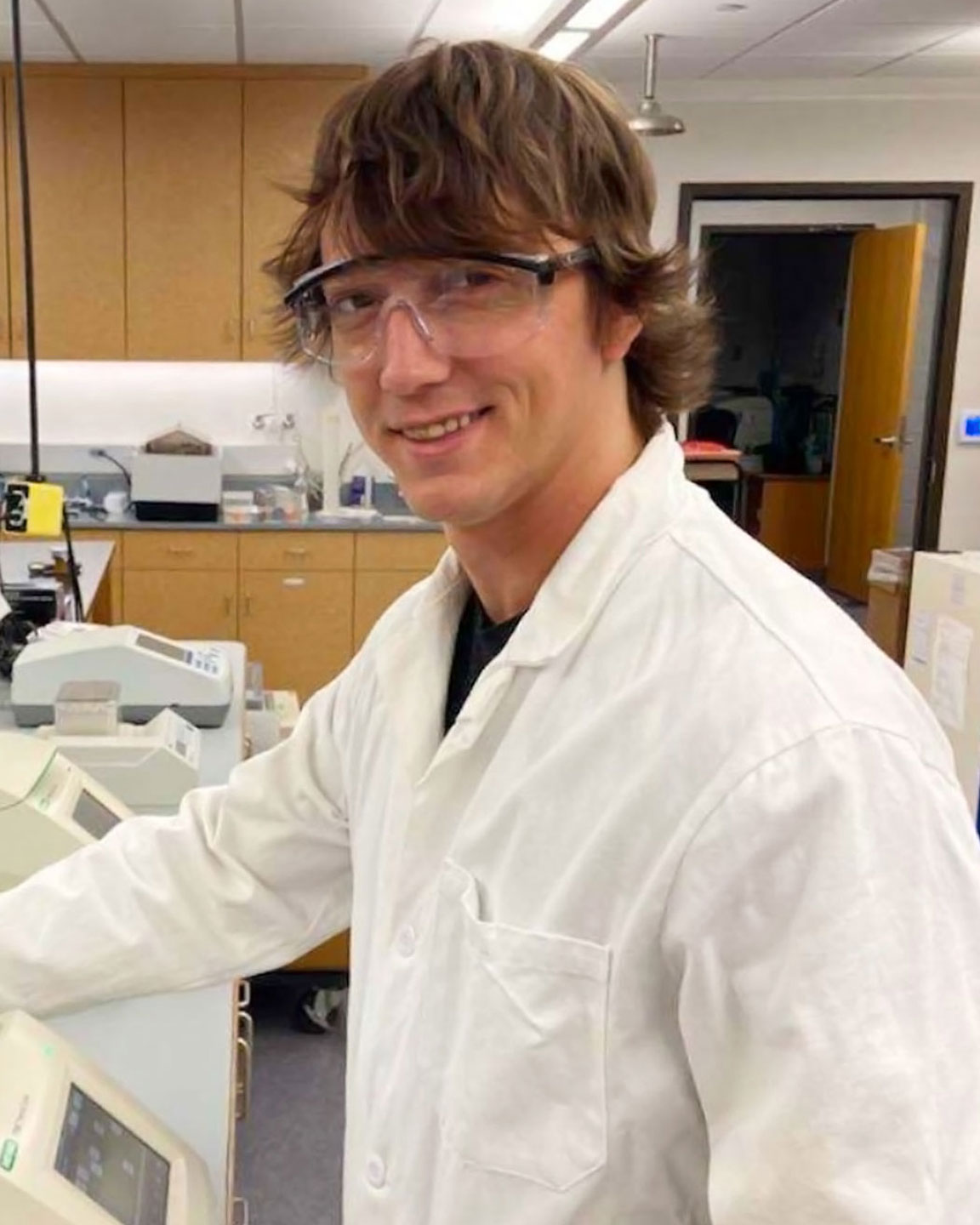
Ty Mellor
Slightly more than 2,000 people currently live in Salina, Utah—just west of a 217,000-acre geological feature called the San Raphael Swell.
It’s a gateway to some of the most remote (and still yet-to-be-permanently settled) land in the Beehive State. But for Carl “Ty” Mellor, it’s been an ideal launching pad for a career in, of all things, microbial engineering. The double-major in chemical engineering and cellular & molecular biology places Mellor on the edge of a different frontier than that of the magnificent badlands of brightly colored and wildly eroded sandstone formations, populated by wild horses and etched drawings from the ancestors of today’s Native Americans.
Frontiers, after all, can be both big … and small. The deep canyons and giant plates of stone tilted upright in his hometown’s backyard are metaphors for the scientific reveals that await the young scientist who, inversely, investigates the micro universe rather than the macro one of massive geologic upheavals where he spent time as a youth camping and hiking with friends.
“In physics,” says Mellor of his time at the University of Utah, “we were going over things that happen at the micro scale which got me interested. It’s all so complex and there’s so much left to discover on how things work at that scale, and there is so much potential for solutions to real world issues.”
Considered a “non-traditional” student, the twenty-eight-year-old U senior graduated more than a decade ago from North Sevier High School in a class of 46. During that time he worked as a dishwasher, then at Little Caesar’s pizzeria with one winter at Brian Head Ski Resort, followed in his final year at an oil change/tire repair shop. Today, he is the recipient of no fewer than seven university scholarships and awards, including the Joseph T. Crockett and the Neil R. Mitchell Endowed Scholarships.
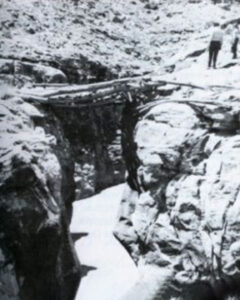
Montell Seely and daughter Fawn examine Swasey’s Leap. Photo: Lee Swasey
From Salina to the bench at one of America’s top research institutions might seem like a leap as far and precipitous as relatively nearby “Swasey’s Leap.” Local legend has it that Sid Swasey bet his brother Charlie that he could jump his horse over the 14-foot wide, 60-foot deep gap which Charlie proceeded to do. But for Mellor, his was a leap clearly worth making. Now embedded in the Kelly Hughes Lab at the School of Biological Sciences, he is busy co-opting the type 3 secretion system used to build flagella in salmonella to secrete proteins of interest and simplify bacterial protein synthesis.
A leap from North Sevier High School, indeed.
When asked to explain something most people don’t known about salmonella, he explains that the pathogenic bacteria is named after Daniel Salmon, the first person in the U.S. to receive a Doctorate of Veterinary Medicine. But, despite his adoration of a pet chihuahua named “Ace,” Mellor won’t be going to veterinary school.
“I think there is a ton of potential [for research] in aging and disease,” he says. “There is so much that we don’t understand yet about the human body. There is also potential in carbon sequestration, either by manufacturing long-term products using carbon or developing microbial carbon sinks that can sink to the bottom of the ocean, for example — possibly being able to manufacture stronger and lighter materials by mimicking the way certain enzymes have incredibly low error rates.”
The last few years have not been easy for Mellor due to the pandemic. But, perhaps surprisingly, he will tell you that he didn’t mind online classes that much. “I was working grave shifts at the time [at the U's Guest House] and was able to watch all of my lectures during downtime at work. Transitioning back to normal life has been much more difficult.”
Difficult or not, in October Mellor jumped right in to share his research poster titled “One Step Protein Purification via the Type 3 Secretion System” at the annual School of Biological Sciences' Science Retreat. His explanations to the curious as well as potentially the friendly combatant-questioner (admittedly rare), was clear, commanding and informed. Poster presentations of this kind are a sort of pay day for an undergraduate: it’s that rare moment when all the hours “at the bench,” under the ‘scope, and under the care of a principal investigator and mentor converge, and one’s scientific findings are distilled into appealing, bite-sized pieces.
As Mellor approaches graduation and graduate studies, he has some advice for his undergraduate cohort: “Keep in touch with old friends and put an effort into connecting to new groups (especially for tough classes). Get lots of fresh air and sunshine, spend some time learning time management, and remember the online skills you had to learn since[,] they’ll always be useful.”
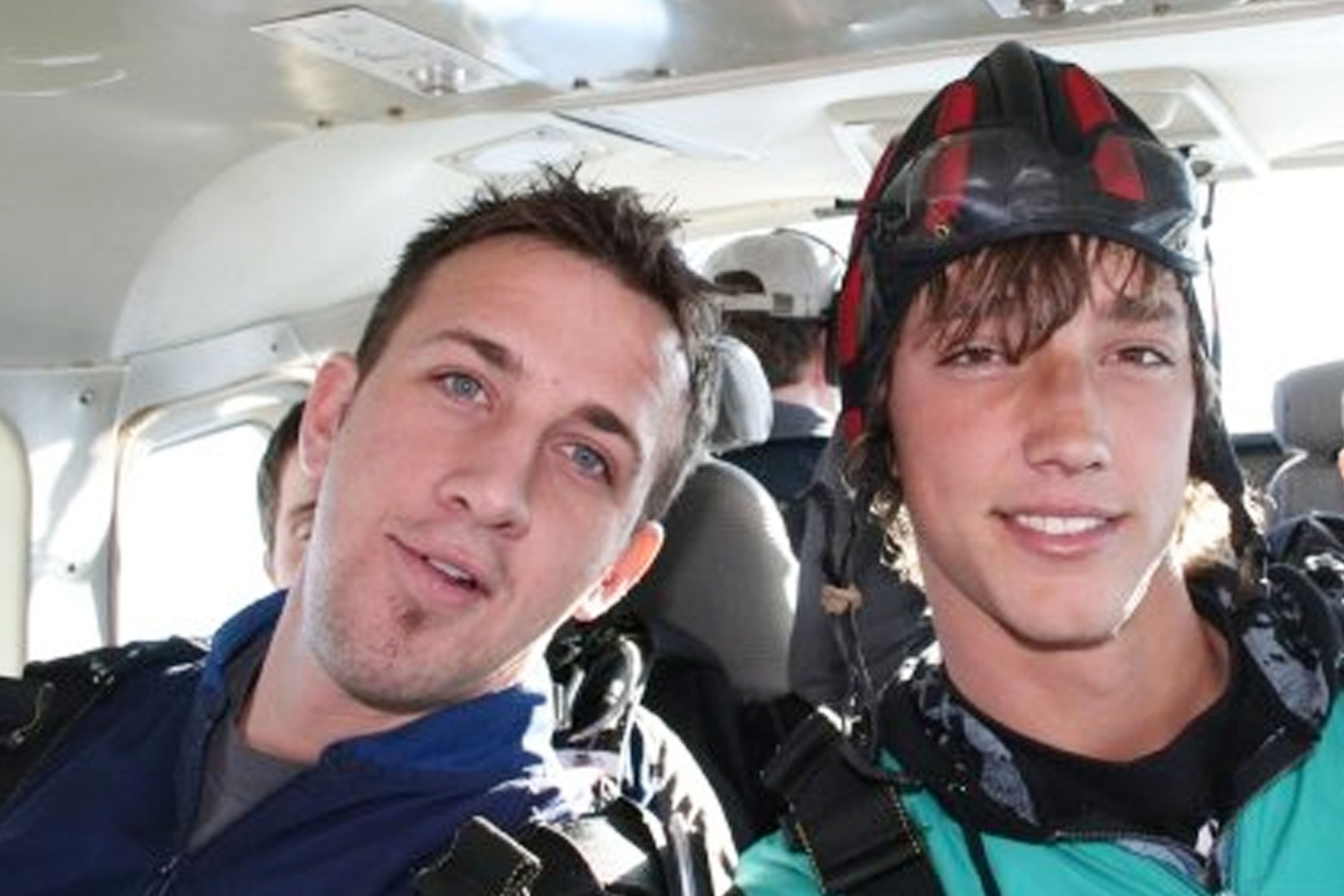
Getting ready for yet another leap, this time out of an airplane, skydiving with brother Casey.
He and his older brother Casey, whom Mellor refers to as his “hero,” still hang out together. “Scientifically, he’s the only one among my family and close friends that I can talk to about research or science in general. Everyone else’s eyes tend to glaze over almost immediately, while he’ll actively argue, ask questions, and come up with his own solutions. We share reading recommendations and talk about any new stuff that pops up in the news … . He’s always been there for me.”
You can take the boy out of Salina but you can’t take the Salina out of the man. And Ty Mellor wouldn’t have it any other way.
by David Pace, first published @ biology.utah.edu.
>> HOME <<
BioKids
BioKids
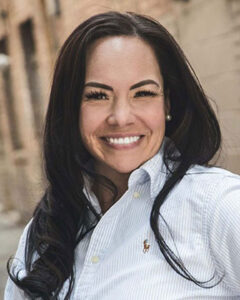
Christine Medina
Earlier this year, when BioKids was awarded a half-million-dollar stabilization grant, where those monies were allocated spoke to the ethic of this celebrated childcare and pre-school at the School of Biological Sciences.
“My first priority was to take care of our staff—to ensure they are receiving equitable wages and benefits,” says Christine Medina, Director. “They are the most critical component to our day-to-day operations. Supporting our staff is the best way to support our families.”
In addition to paying back the College of Science for the building remodel (2020) and and a recent remodeling of its infant/toddler playground, the pandemic relief funds issued by the Office of Childcare: Department of Workforce Services allowed Medina to allot more of her annual budget towards base salaries/wages. As a self-sustaining (recharge) program at the University of Utah, BioKids is required to bring in what it pay outs. And, of course, parents have limits on what they can pay in tuition for their child care costs. “Given the recent workforce demands for increased pay,” Medina says, “the grant has enabled us to meet those demands with most increases around 30% and without further burdening parents.”
This innovative ethic first underscored the launch of BioKids in 1999 when a group of biology faculty decided they wanted to make it easier and more convenient for faculty to care for their young children during the work day. As a parent cooperative program, Medina explains, “parents are involved in the program and encouraged to participate in daily activities and needs of the classrooms. Parents and Staff work together.”
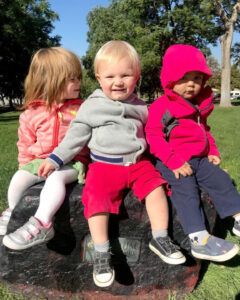
The three amigos.
In the early days, faculty took turns teaching the children and directing the program outside of their positions in the Biology Department (now the School of Biological Sciences). “It was a grass roots effort to provide childcare,” says Medina, and then quips with a smile, “Dave Gard, a cell biology professor [now emeritus], operated BioKids as the Director for a short while and said, ‘it was the worst job I ever had.’” Then when the pandemic happened, and high-touch parental involvement had to end.
Clearly, a new model was needed. Today, in addition to Medina as director, there are 13 employees that thread through three classrooms each hosting a different age range. The three-class model supports the current research for how children learn and develop: infants (3 months to 18 months); toddler (18-33 months) and pre-school (30 months to 5 years old).
What makes BioKids with an enrollment of 40-45 children at any given time distinctive from other day care/pre-schools on campus?
“Most faculty and staff can see their children from their offices/labs while outside on walks or on the playground. Or, they are [just] a short walk away. Many of their colleagues are also enrolled, and their children are spending time creating friendships and bonds that span the family,” says Medina.
In addition to the close proximity of BioKids, housed in Building 44 just south of the Skaggs Biology Building, each cohort of children has a low enrollment with low student/teacher ratios. This allows staff to get to know each individual and plan a “whole child” approach to their learning. The more professional staff also means that children’s progress and comprehension are monitored with developmental assessments and portfolios. BioKids is now accredited by the National Association for the Education of Young Children, representing early childhood education teachers, para-educators, center directors, trainers, college educators, families of young children, policy makers, and advocates.
Another advantage to the BioKids model is that children move up and progress through classrooms based on their age and the designated age range of each class. Children enroll based on the parents’ need, generally for a 4-to-5-year span and without pause. The child care/pre-school has a reputation as one of the most successful and desired programs in the city. No wonder there’s a three-year wait for families outside of SBS and the College of Science which have priority status in admissions.
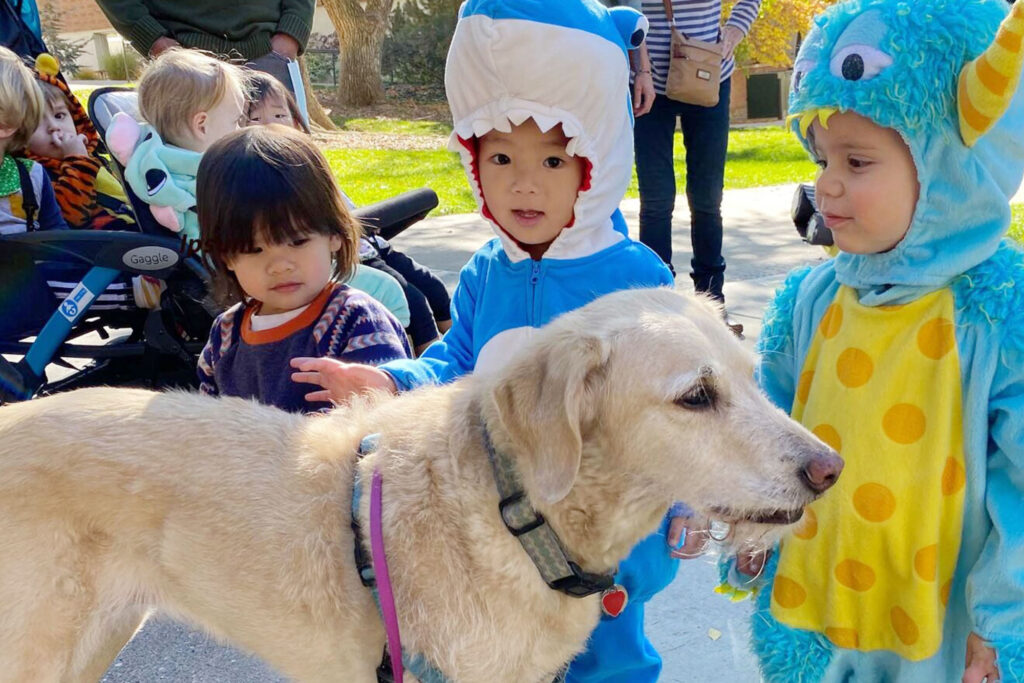
Trick-or-treating on campus.
Parents are not the only ones who appreciate the kind of continuity in their child’s life . . . as well as their own . . . that BioKids affords. Staff are clearly happy as well. Turnover is “very low,” something that a 30% raise in wages is likely to further cement. Remarkably, “both original preschool teachers hired in 1999 are still with us today,” says Medina. “Their first hoard of children are now in college!”
As for Medina, she has been an administrator for prominent early childhood programs in Salt Lake for 23 years and serves on several committees throughout the state. There she advises on state-level policies and initiatives for the childcare industry—for both the child and the worker. Her undergraduate degree is in Family and Human Studies, and she also holds a National Administrators Credential and a Child Development Associates credential, along with several state endorsements.
It’s easy to see why BioKids is such a hit. Outside both main biology buildings (South Biology and Skaggs) the ambient noise of childhood play wafts in on any given day, and the ritual of parents coming and going to drop off and pick up their wee ones is heartwarming. Every Halloween (especially now that the pandemic has eased some) a trail of children costumed as lizards (or are they dinosaurs?) and other life forms, arrive at the Main Office to trick or treat and endure comments like, “Oh, wow, the freshman are getting younger every year!”
But it is the daily routine that is most charming. Markus Babst, Associate Professor of Biology and Director of the Cell & Genome Center is all smiles every morning when he drops of his two-and-a-half-year-old at the historic building built during World War II with original windows, moldings, hardware and exposed brick. (The building was originally the student health facility.) A first child, six-year-old Oskar, is already a BioKids graduate, and this second child, Mari, along with her parents are more than happy to have Mari enrolled in what Babst calls “a warm, friendly and educational place.”
End of day, Babst returns on his commuter bike towing its requisite canopied trailer for toddlers, and will sometimes lift his daughter over the chain-link fence to give her a hug, strap her in and then head for home. It’s a bucolic scene right here on campus, usually commandeered by college pursuits, and it spurs passing students to look up from the perpetual viewing of their mobile screen and . . . smile. Sometimes they even stop and watch for a few moments perhaps remembering something nostalgic about their own past as a small person.
by David Pace, first published in biology.utah.edu.
Stephanie VanBeuge
Stephanie VanBeuge
Lockdowns are something that Stephanie VanBeuge BS’17 knows something about–even before the pandemic.
It was in her third year of graduate school at the University of Oregon when VanBeuge was first diagnosed with brain cancer–on the first day of the school year. She returned to Utah to receive treatment at Huntsman Cancer Institute and was able to return to school almost like nothing ever happened.
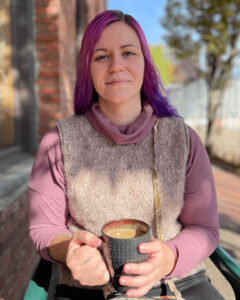
Stephanie VanBeuge
“When the pandemic started, I had just finished radiation treatment for my brain cancer. For about four months before lockdown started in March 2020, I was on my own lockdown of sorts recovering from brain surgery and enduring radiation."
Adjusting to the isolation of the early days of the pandemic was easy enough, she admits, “but starting to work from home and then going back into the lab later that year was really difficult, in part because my brain just wasn’t working like it used to. It’s hard for me to gauge how hard the pandemic specifically has been because as I’ve adjusted to the pandemic I’ve also recovered from brain cancer and, as my brain has continued to heal, I’ve had an easier time navigating our ‘new normal.'”
The U, VanBeuge says, gave her a lot of confidence in exploring new topics. “I chose to rotate in labs that were different from the kind of research I had done before. I was able to learn a lot about myself and my interests as a scientist and make an informed decision on my degree.” That was a good thing, because in Oregon students rotate through three labs during their first year and then pick one of those labs in which to work on their PhD. VanBeuge chose Karen Guillemin’s lab where she studied host-microbiome relationships.
Now with her doctorate, VanBeuge, who is originally from Tacoma, WA but grew up in Las Vegas, is looking to start a career in the biotechnology industry. “I was interested in the evolutionarily conserved aspects of this relationship and focused on gut epithelial proliferation in response to colonization by the microbiota.” During her research she found that the multiplication or reproduction of epithelial cells which in the expansion of a cell population (epithelial proliferation) wasn’t a response to a specific bacterial species. Instead, “it’s an innate immune system mediated response to barrier damage.”
Along the way VanBeuge has been active in the University of Oregon Women in Graduate Sciences (UOWGS) - https://twitter.com/uowgs organization where she served as outreach chair for AY 2019-2020. Her research culminated in two papers that she co-authored, “Proteolytic Degradation and Inflammation Play Critical Roles in Polypoidal Choroidal Vasculopathy” in The American Journal of Pathology and “Secreted Aeromonas GlcNAc binding protein GbpA stimulates epithelial cell proliferation in the zebrafish intestine” in bioRxiv. A third paper has also been submitted.
Reporting on her research is just one writing outlet for Stephanie VanBeuge. She’s determined to produce a memoir of what it was like as a young scientist, battling brain cancer in the middle of her education. She has a first draft and plans on completing it soon. The story “is primarily a story about resilience. It’s about facing your fears and uncertainty head on and not letting them stop you from showing up and fighting back. I hope people who read this book are empowered to show up and face their own challenges head on.”
By David Pace, originally published at of biology.utah.edu.
Are you a Science Alumni? Connect with us today!
Stolen Ivory
Stolen Ivory
Isotope data strengthens suspicions of ivory stockpile theft.
In January 2019, a seizure of 3.3 tons of ivory in Uganda turned up something surprising: markings on some of the tusks suggested that they may have been taken from a stockpile of ivory kept, it was thought, strictly under lock and key by the government of Burundi.
A new study from University of Utah distinguished professor Thure Cerling and colleagues, published in Proceedings of the National Academy of Sciences, uses carbon isotope science to show that the marked tusks were more than 30 years old and somehow had found their way from the guarded government stockpile into the hands of illegal ivory traders. The results suggest that governments that maintain ivory stockpiles may want to take a closer look at their inventory.
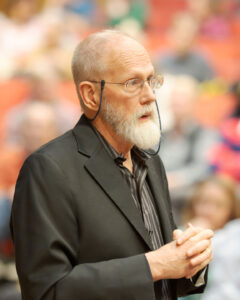
Thure Cerling
“Due to the markings seen on some samples of the ivory, it was thought that quite a few samples in this shipment could be related to material held in a government stockpile in Burundi.”
Ivory’s isotope signatures
Cerling is a pioneer in the use of isotopes to answer questions about physical and biological processes. “Isotopes” of a given element refer to atoms of the element that vary in their number of neutrons, and thus vary oh-so-slightly in mass. A carbon-14 isotope has one more neutron than carbon-13, for example.
Some isotopes are stable and some are unstable. Unstable isotopes decay into other isotopes or elements through radioactive decay. Since the rate of decay is known for unstable isotopes, we can use the amounts present in a sample to determine ages. That’s how carbon dating works—it uses the rate of decay of unstable carbon-14 to determine the age of organic matter.
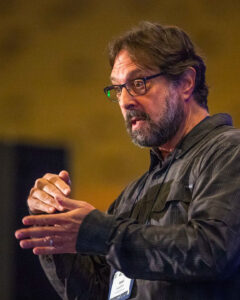
Sam Wasser
Around a decade ago, Cerling attended a presentation at the U by Sam Wasser of the University of Washington, who was studying the genetics of wildlife and using those tools to investigate the date and place of wildlife poaching. Cerling, recognizing that his expertise in isotope science might be able to add useful information, began an ongoing collaboration with Wasser.
In 2016, Cerling, Wasser and colleagues published a study that addressed a key question in the ivory trade: how old is the ivory seized by governments? Some traders have claimed their ivory is old, taken before 1976, and thus exempt from sales bans. And with the average size of ivory seizures more than 2.5 tons, researchers, governments and conservationists wonder how much of the ivory is recent and how much is coming from criminal stockpiles—or is stolen from one of several ivory stockpiles held by the governments of some countries in Africa.
“Governments keep their stockpiles for multiple reasons,” Wasser says. “They hope to sell the ivory for revenue, sometimes to support conservation efforts. However, they can only sell ivory from elephants that died of natural causes or were culled because they were problem animals. They can’t sell seized ivory because they don’t know it came from the country.”
With the combination of Cerling’s isotope data and Wasser’s genetic data, the 2016 study found that more than 90% of seized ivory was from elephants that had been killed less than three years before. It was a sobering result, showing active and well-developed poaching and export networks. The study seemed to show that little ivory from government stockpiles had ended up on the black market.
Marked tusks
But the 2019 seizure of ivory in Uganda showed something concerning. Some of the tusks sported markings that looked suspiciously like the markings that CITES, the Convention on International Trade in Endangered Species of Wild Fauna and Flora, uses to inventory stockpiled ivory.
Due to the markings seen on some samples of the ivory,” Cerling says, “it was thought that quite a few samples in this shipment could be related to material held in a government stockpile in Burundi. We were asked to date samples from this, and three other recent ivory seizures, to see if some samples could possibly be from older stockpiles.”
To determine the ivory’s age, the researchers collected small samples from the tusks and analyzed them for the amount of carbon-14 isotopes in each sample. They were looking specifically for the amount of “bomb carbon” in the tusks. Between 1945 and 1963, nuclear weapons testing doubled the amount of carbon-14 in the atmosphere, so anything living that’s consumed carbon since then—including you—has a measurable carbon-14 signature. The amount of carbon-14 in a sample of ivory that hasn’t yet radioactively decayed can tell scientists when the ivory stopped growing, or when the elephant died.
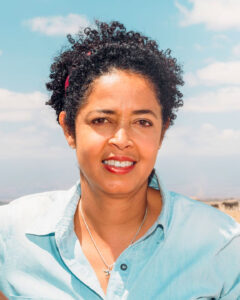
Paula Kahumbu
The method takes some calibration, using samples from organisms living in the same area. Some of the samples came from schoolchildren in Kenya, through a program called “Kids and Goats for Elephants.” Because most families in rural Kenya keep goats the program, run by Cerling and Paula Kahumbu of WildlifeDirect, engages children in collecting hair samples from goats for isotopic analysis. The isotope data is useful for many applications, including fighting elephant poaching and, in this case, calibrating the bomb carbon decay rate for more accurate dating of ivory.
A consequential result
The researchers analyzed ivory from four seizures in Angola, Hong Kong, Singapore and Uganda. Genetic data ensured that they weren’t sampling two tusks from the same individual. The results of analysis from the Angola, Hong Kong and Singapore seizures were as expected – the results showed ages mostly around three years after the death of the elephant, with no tusks having been taken more than 10 years previous.
But the Uganda seizure, with the inventory markings on the tusks, showed something very different. Nine of the 11 tusks tested had been taken more than 30 years before, with the dates of death ranging between 1985 and 1988. Those dates are consistent with the age of ivory in the stockpile of the government of Burundi, which was inventoried and stored in sealed containers in 1989.
“My suspicions were affirmed,” Wasser says. “The bigger surprise was how near to 1989 the elephants were killed.” At the time Burundi assembled its stockpile, a condition of joining CITES, which assists governments in managing ivory reserves, was that the ivory to be stockpiled was old. The results suggest that that wasn’t the case, Wasser says, which would have violated conditions for Burundi to join CITES.
“The hope is that CITES will request the stockpile to be re-inventoried,” Wasser says, “including aging randomly selected tusks and secure the remaining stocks.”
Find the full study here.
by Paul Gabrielsen, first published in @theU.
Hedgehog Signaling
Hedgehog Signaling
A cracker jack team of U of U undergrads works with principal investigator Ben Myers to break open a decades-old biological mystery of Hedgehog Signaling.
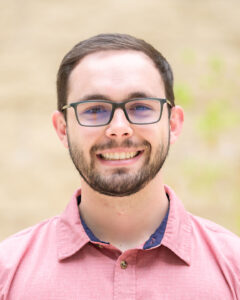
Corvin Arveseth
Corvin Arveseth, BS’21, can’t remember when he wasn’t fascinated by science and biology. So, when he came to the University of Utah and declared his majors in biology and biochemistry, he knew he wanted hands-on experience in research. “I didn’t know anything [about the] Hedgehog (Hh) signaling [pathway] until I read an advertisement put out by Ben Myers, [principal investigator at Huntsman Cancer Institute, assistant professor of oncological sciences at the University of Utah, and head of the Myers Lab] in a biology department newsletter looking for undergraduate researchers,” he says. “After reading some background information and meeting with Ben about the Hh pathway, I became intrigued with the work being done in his lab.”
The Hh pathway he’s referring to is akin to a master set of instructions for animal development and regeneration. It controls the formation of nearly every organ in the human body. Signaling pathways like Hh serve as molecular “telephone wires” from the cell surface to the nucleus. When cells in our bodies communicate with one another, signals are relayed along these molecular telephone wires, turning on expression of genes involved in growth, differentiation, or in some cases skin and brain cancers.
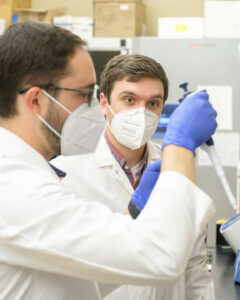
Corvin Arveseth and Will Steiner
The Hh pathway got its unusual name from decades-old genetic studies in fruit flies, where mutations in critical developmental genes led the flies to take on a bristly hedgehog-like appearance. However, versions of the Hh pathway operate throughout the animal kingdom, controlling development, stem cell biology, and cancer in many different contexts.
But even after many years of effort by labs all over the world, surprisingly little was known about how the Hh pathway actually works at a molecular level. Scientists knew that the signals conveyed by these molecular telephone wires were fundamental to human development and disease, but they didn’t know what the signals were, or how they were transmitted intracellularly. Consequently, health researchers’ ability to control Hh signaling in many diseases including cancer had been limited.
So, this is a story not just about a seemingly intractable research question, which is de rigeur in scientific circles, but how a team of largely undergraduate students in a four-year-old lab worked together under enormous odds to shake loose that answer. Myers says that that it was because of inexperience, not in spite of it, that the undergraduates in his lab were able to make these discoveries. These students’ fresh, undaunted determination to scientific inquiry, combined with a lack of preconceived notions and a willingness to learn, were key factors that enabled their groundbreaking discoveries.
Two papers, both with U undergraduates as first or co-first authors, were the gratifying result. PLOS Biology and Nature.com
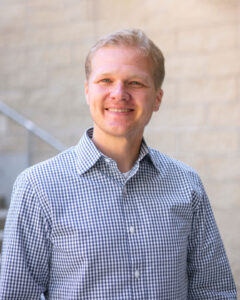
Ben Myers
“It is a truly remarkable and inspiring collaboration that continues to this day, and I am so proud of how everybody was able to join forces and overcome so many obstacles created by the COVID-19 pandemic.”
Mysterious pathways
When Myers first set up his lab at the U in 2018, the key molecule in the Hh pathway that grabbed his attention was SMOOTHENED (SMO), a so-called “transmembrane protein” that spans across the cell membrane from the outside to the interior. SMO was known to be critical for transmitting signals from the cell surface to the nucleus. But what were the five or six steps between receiving the message and turning on gene expression? There was a “major disconnection about how this worked,” says Myers.
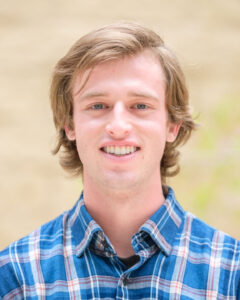
Nate Iverson
The twenty-five-year-old mystery was indeed tantalizing. It was “this interesting mystery coupled with the importance of Hh function,” says Arveseth, “in developmental and cancer biology [which] hooked me right away.”
Spearheading the project
Arveseth was the point of the spear for this project begun at the beginning of his sophomore year. But there were many others on the team, all of whom are “both incredibly smart, and also very kind and a lot of fun to work with,” according to Myers.
This includes Nate Iverson, a third year chemistry major with an interest in cellular signaling. “Having HCI in close connection with the University gave me greater access to research possibilities, and I was able to find an opening in the Myers lab studying Hh signal transduction.”
And then there was biology major Isaac Nelson, who worked tirelessly to produce a freezer full of carefully prepared, purified fragments of SMO for biochemical studies, only to hit a brick wall when he and Myers were unable to formulate a good hypothesis to drive an experiment.
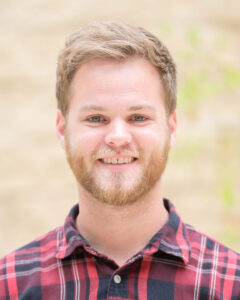
Isaac Nelson
“It was only after starting up an international collaboration,” says Myers, “that the critical experiments snapped into view for us.” This led Nelson to send his samples to one of the lab’s new collaborators in Germany, and they used his samples to try an experiment that worked right away. In the midst of a raging pandemic, Nelson’s purified proteins helped to launch a new and entirely unexpected phase of the project, expanding the collaboration to include other scientists around the world.
“It was another scenario,” says Myers, “where everyone worked well together.”
Recent graduate Madison “Madi” Walker, BS’21, with a cell and molecular emphasis, was also part of the team. She is still working in the Myers lab studying another critical aspect of SMO signaling, namely the interaction between SMO and the enzyme G protein-coupled receptor kinase 2. Earlier, former undergraduate Jacob Capener, BS’20, assisted in the work.
Another critical member of the Myers lab team is Will Steiner, BS’21, who is currently collaborating with Arveseth and Nelson to purify SMO in complex with its binding partners in order to work out their atomic structures. He became interested in this area of research after taking the cell biology and biochemistry course at the U. “Biochemistry was particularly compelling and got me excited about the chemical reactions behind human physiology,” he says.
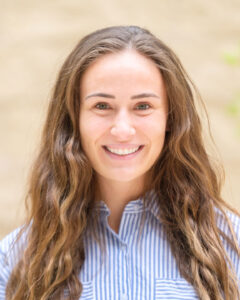
Madison Walker
It starts in the classroom
Rigorous courses were critical in preparing Myers’ undergraduate team for the hands-on research that led to their remarkable findings in the lab. He has nothing but kudos for the U’s curriculum. “Coursework before the lab experience [for undergraduate researchers] was very, very good here. In general, I’ve been lucky to attract motivated and curious students to my lab. They are inspired to push the research forward. They are all up to the challenge. And they have a great esprit de corps. They all work incredibly well together as a team to drive the science forward.”
That kind of correlated teamwork was not necessarily easy to enact under the circumstances. “Fortunately, we were able to finish the last key experiment of the first paper,” says Myers, in March 2020, just before the pandemic started to take hold and shut lab work down. He’s always believed that having undergraduates get a taste of cutting-edge research is important. They “shouldn’t have to work on something trivial… . What’s exciting about science is to push the boundaries.”
And yes, for Myers and the other senior members of his lab, including graduate students Danielle Hedeen and Aram Centeno, lab manager Ju-Fen Zhu, and former lab technician John Happ, “you have to be committed to helping everybody in your lab, even if they’re neophytes.” Clearly it’s been worth it. “And being a little bit of a neophyte is good,” he says, “because you don’t talk yourself out of doing experiments that are simple, unorthodox.”
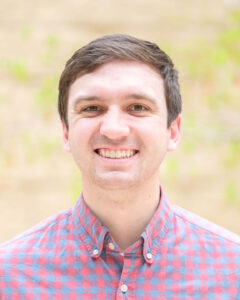
Will Steiner
Asking the right questions
What Myers is trying to say, and seems to have proven over the course of the past three years and now the publication of two discovery-laden papers, is that their remarkable findings stemmed from the initial naïve view that the SMO protein didn’t fit the mold of other proteins as was previously assumed. He and Arveseth took a guess that SMO might be directly coupled to a critical intracellular signaling molecule called PKA. This was a rather wild idea, since there were few if any examples of transmembrane proteins that directly interacted with PKA. “It was a guess, how it might work, and a couple of months later: big discovery. Our initial guess was on the right track. There was a whole new unexpected thing going on but that made sense.”
Though early on the team suspected what they had discovered was important, “we didn’t know if we had a full explanation of how the system worked. We weren’t sure if it was the main event or an auxiliary event.” In the first paper, published in the journal PLOS Biology last year, they explained that: what they thought they knew, and what they weren’t sure about . . . yet.
But it was only after the pandemic was in full force that the team pivoted to the second exciting phase of the project, expanding to include Susan Taylor’s lab at the University of California, San Diego, one of the world’s foremost authorities on the PKA molecule the Myers team had implicated in their research.
Taylor and her colleagues had a critical insight regarding the SMO-PKA interaction which eventually formed the basis of a second manuscript, recently published in Nature Structural and Molecular Biology. “It is a truly remarkable and inspiring collaboration that continues to this day, and I am so proud of how everybody was able to join forces and overcome so many obstacles created by the COVID-19 pandemic,” says Myers. And his team is anticipating that even more exciting discoveries are on the horizon. Eventually, this work may lead to better drugs to treat some of the diseases that result from aberrant Hh signaling, including various skin and brain cancers.
In all, with the resulting two papers, the project turned out to be a “best case scenario that wasn’t planned,” and a lesson of how important it is to keep an open mind, which often leads to big discoveries.
Success is never final, however. And Arveseth, recipient of no less than ten scholarships and awards during his sojourn at the U, is now enrolled in the MD/PhD program at the Washington University in St. Louis, where he will focus on hematology and oncology. His colleagues are also pursuing their academic and research careers full-steam ahead. They, along with their mentor, Ben Myers are a testament to the notion that persistence in knowledge gathering pays off but that it must be paired and even driven by a relentlessly open mind.
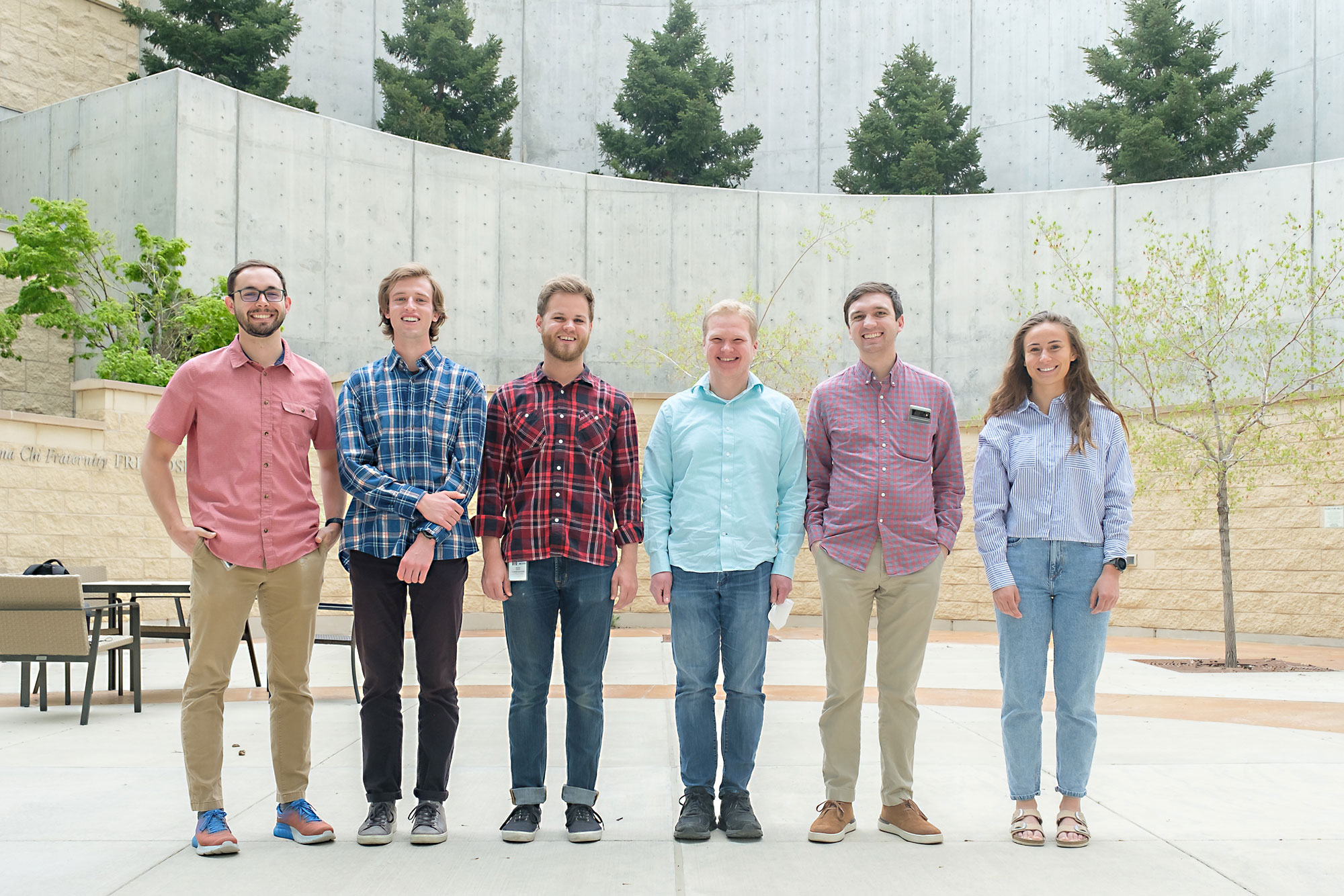
The Meyers Lab
Concludes Myers, “To be honest, it comes down to the willingness to try new things and to have the ability to work together as a team. In reality, this would have been way too much for any individual scientist, even a highly trained one, to do alone.” You can follow him and his lab on Twitter @Myers_lab
Find the full study here.
by David Pace, first published @ biology.utah.edu.
Ethiopian Abattoirs
Ethiopian Abattoirs
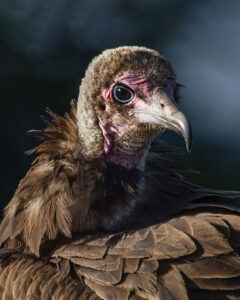
Hooded Vulture
The decline of vultures and rise of dogs carries disease risks.
In the yards behind the slaughterhouses—also called abattoirs—of Ethiopia, an ecological shift is unfolding that echoes similar crises the world over. Species with a clear and effective ecological role are in serious decline, and the less-specialized but more aggressive species that have moved in to take their place are not only less effective, but are harmful to their ecosystem which, in this case, includes humans.
This is a story about vultures, feral dogs, rabies—and piles of rotting animal carcasses. Buckle up. But in the end, it’s about the power of conservation to keep ecosystems, even urban ecosystems, in balance, benefitting the people who live there.
“Carrion consumption by vultures is declining, and increasing by most other scavengers, but that increase is not sufficient to make up for the loss of vultures.” says SBS alumnus Evan Buechley, PhD’17, now with The Peregrine Fund, “So there’s a gap there. And what happens with that gap is a bit of an unanswered question, but that’s where the problem lies.”
The study is published in the Journal of Wildlife Management and is funded by the National Science Foundation, the University of Utah, HawkWatch International, The Peregrine Fund and the National Geographic Society.
Vultures are awesome
Worldwide, vultures are perfectly equipped to take care of the unpleasant remnants of death. Rotting carcasses can become hotbeds of disease, overrun by bacteria and insects. But vultures are an efficient clean-up crew. By eating carrion, they remove the carcasses and pass them through a highly acidic digestive system that wipes out disease-causing agents. And a diversity of vultures is better—some species are specialized to tear away hides and skin while others, coming in last, literally gulp down the bones.
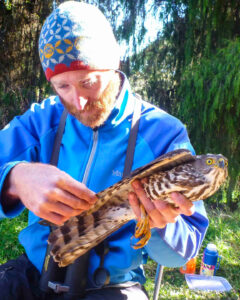
Evan Buechley
“Carrion consumption by vultures is declining, and increasing by most other scavengers, but that increase is not sufficient enough to make up for the loss of vultures.”
But vultures have been in trouble in recent decades. They’re susceptible to poisons in the carrion they eat, whether that’s lead ammunition, the drug diclofenac, or poisons used against predatory animals. And with vultures producing relatively few chicks and taking a relatively long time to mature, it’s harder for them to recover from population declines.
Çağan Şekercioğlu, associate professor in the University of Utah School of Biological Sciences, showed that vultures were the most threatened group of birds (called an ecological guild, when the group uses the same or related resources) in 2004 when he conducted the first known ecological analysis of all bird species while in graduate school.
In 2012, Şekercioğlu accepted Buechley as his first doctoral student at the U. Buechley brought extensive experience working with vultures and condors. He and Şekercioğlu began a project tracking Egyptian vultures in eastern Turkey and the Horn of Africa.
“Evan led this project brilliantly and expanded it to the other vulture species of Ethiopia and the Horn,” Şekercioğlu says. “Despite the many challenges, he also decided to study the scavenger communities of the Addis Ababa abattoirs, to quantify the causes and consequences of vulture declines in the region.”
In 2016, Şekercioğlu and Buechley re-analyzed the ecology of all bird species. “We realized that vultures not only have the fewest species of any avian ecological guild, making them irreplaceable, but since that first analysis in 2004, they had gone downhill faster than any other group,” Şekercioğlu says.
Yes, there are other scavenger species that can take vultures’ place at the carrion table. But the loss of vultures, as we’ll see, can lead to human costs.
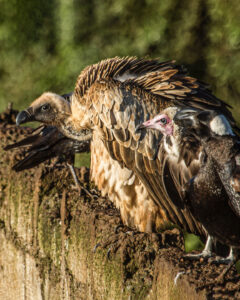
A white-backed vulture, a hooded vulture and a thick-billed raven.
Abattoirs’ feathered “employees”
At the abattoirs of Ethiopia, vultures are welcome partners. After butchering animals in clean conditions, the workers move the remnants of the carcasses – hooves, organs and bones, for example, to separate compounds. It’s a . . . unique sensory experience, Buechley says.
“It can be pretty stinky and pretty gross, by any objective measure.”
So abattoirs are grateful for the scavengers, including critically endangered white-backed, Rüppell’s and hooded vultures, that eagerly clean up the pile.
Study co-author Alazar Daka Ruffo, from Addis Ababa University, has interviewed abattoir staff members to see how they feel about the vultures.
“Some abattoir staff say half-jokingly, but not fully, that they see the vultures as employees of the abattoir,” says Buechley, reporting Ruffo’s findings. “They’re serving an important function. There’s intentionality behind the system.”
Other winged scavengers frequent the disposal piles, including crows, ravens, ibises and marabou storks. Four-legged visitors include packs of feral dogs.
“It’s an urban ecology situation where you have the human food supply meeting and really directly interacting with the wildlife food supply of scavengers,” Buechley adds. “It’s just a really complicated, kind of gross but fascinating system.”
With a research team including Rebecca Bishop, Tara Christensen and Şekercioğlu from the U’s School of Biological Sciences, Buechley set out to quantify the amount of carrion consumed by scavengers at six abattoirs in Ethiopia over five years, from 2014 to 2019.
Decline in vultures and rise in rabies
The team noted the types and abundance of scavengers that visited the abattoir buffets, and used this to extrapolate how much they ate. At first, vultures were eating more than half of the carrion in the disposal piles. White-backed, Rüppell’s and hooded vultures together ate an average of around 550 pounds (250 kg) of carrion a day.
But by the end of the five-year study, the number of Rüppell’s and white-backed vultures visiting the abattoir disposal yards decreased by 73%. Hooded vulture visits decreased by 15%. Over the same time, feral dog detections more than doubled.
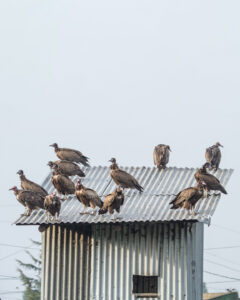
A committee of hooded vultures.
“Although we can’t say for sure if the decline represents a population crash or if the vultures are being displaced by dogs and moving away from the abattoirs, either way this is really concerning,” says Megan Murgatroyd, Interim Director of International Programs for HawkWatch International.
“We know that the vultures are declining and we know that the feral dogs are increasing, but we don’t know exactly why,” Buechley says, adding that abattoir practices are also changing and that further studies will be needed to draw a cause-and-effect relationship.
Regardless, the vultures can ill afford the loss of abattoirs as a food supply. Rüppell’s, white-backed and hooded vultures are listed as critically endangered. “That’s the highest threat category before going extinct or extinct in the wild,” Buechley says.
The population of Rüppell’s vultures has declined by over 90% over the past three generations (approximately 40 years). White-backed and hooded vultures are doing a little better—but not by much. They’re estimated to have declined by 81% and 83%, respectively, over three generations.
“So it does seem that their disappearance from abattoirs is likely linked to a population crash,” says Murgatroyd. “Vultures need all the help they can get right now, and having to compete with growing dog populations is only making things worse.”
Other scavengers on the rise, including dogs, ibises and corvids (crows and ravens) couldn’t pick up the slack at the abattoirs. By 2019, scavengers were consuming nearly 43,000 pounds (around 20,000 kg) less carrion per year than they were in 2014, back when vultures were more abundant and dogs more scarce.
A chilling consequence of the rise of dogs may be a rise of rabies rates in humans. In the late 1990s, vulture populations in India and Pakistan crashed. Feral dog populations increased to take advantage of the uneaten carrion.
“They’re also disease vectors,” Buechley says, “and they interact really closely with people. And there’s been a link drawn between a big spike in feral dog populations and rabies in India.”
Is the same thing likely to happen in Ethiopia? Scientists haven’t yet drawn a link between vulture loss and rabies rise in that country. But Ethiopia already bears a heavy rabies burden with around 3,000 deaths from the disease per year.
“Unlike a lot of diseases which impact the elderly, rabies disproportionately affects young children, which are the most likely to be bit by rabid dogs,” Buechley says.
Fencing dogs out
The researchers provide a straightforward recommendation to help the situation: Use fences to keep the dogs out. And many abattoirs already have fences in place.
“But a pack of feral dogs is really persistent,” Buechley says. “It’s hard to keep hungry animals away from lots of food.”
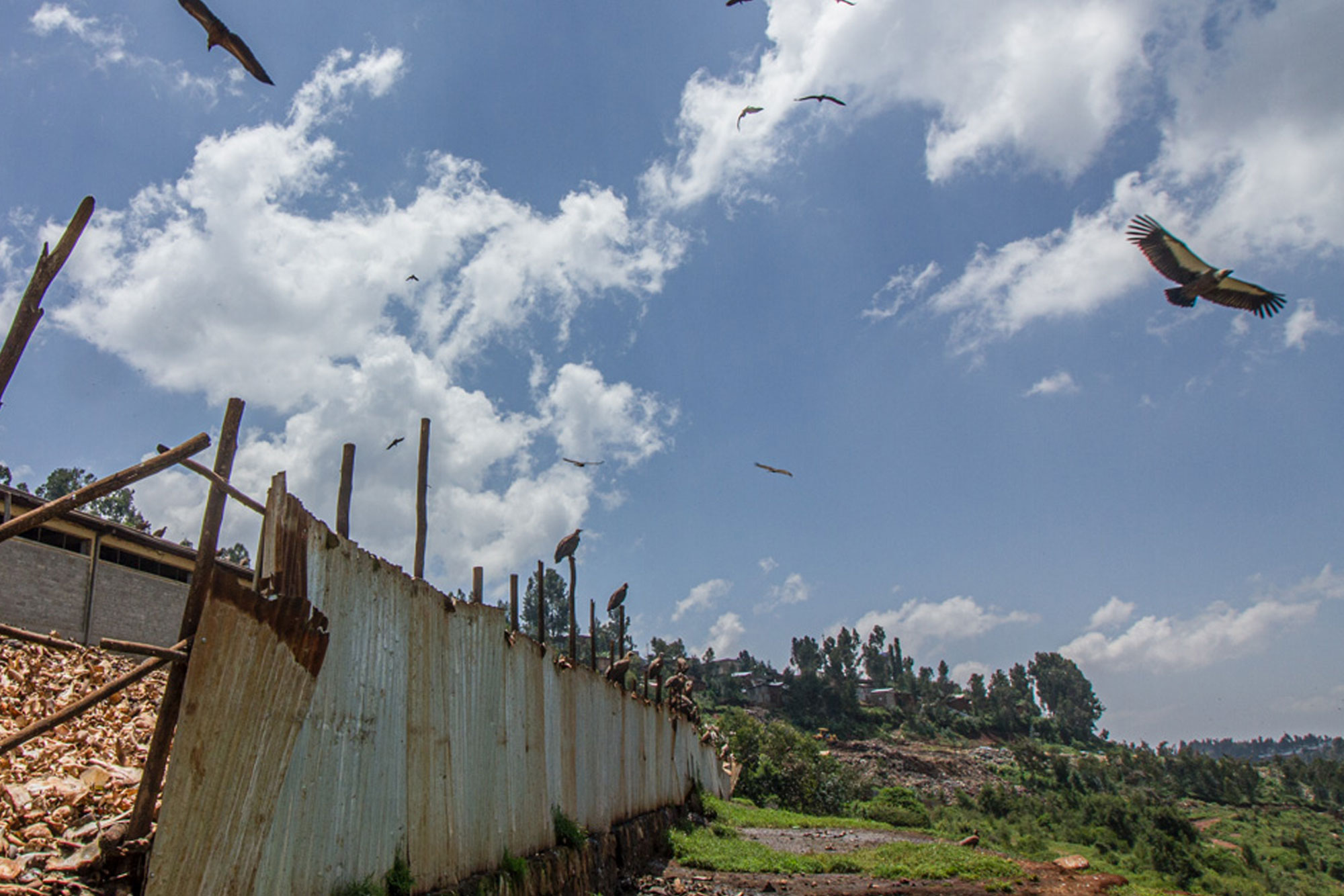
An abattoir disposal pile with a kettle of vultures overhead.
The dogs can fight and dig their way through many fences, and maintaining or fortifying them may cut into the abattoirs’ profit margins.
“It’s a matter of weighing how important it is to keep the fences maintained,” Buechley says. “Improvement of these fences could really have a lot of benefits.” Those include potentially reducing the numbers of feral dogs, which reproduce quickly and whose population keeps pace with the available food supply. That in turn could help control rabies in humans and diseases in other animals, such as the critically endangered Ethiopian wolf, which are carried by the feral dogs.
And, counterintuitively, fencing out the abundant dogs could increase the rates of carrion consumption. Without the dogs around to scare off other scavengers, vultures could return in larger numbers to more quickly and efficiently clean up the disposal piles.
“That could lead to less smell, less groundwater contamination, fewer insects like flies that can breed on the carcasses,” Buechley says. “There’s a lot of potential benefits of investing in repairing the fences around abattoirs, which are found throughout Africa and elsewhere worldwide. We encourage abattoirs, local governments and international organizations to consider this when looking for solutions to waste disposal, human health and scavenger conservation.”
The results of the study show that the loss of specialist species from an ecosystem can’t always be compensated for by other species.
“The overarching point is that vultures are super important,” Buechley says. “If they decline, we expect there to be pretty profound ecological consequences and there may be increases in human disease burden. And so we should appreciate vultures and invest in their conservation.”
Find the full study here.
by Paul Gabrielsen, first published in @theU.
Visualizing the Infinitesimal
Visualizing the Infinitesimal
Even before Andreas Vesalius (1514-1564) first put pen to paper to draw the human form in anatomical detail, scientists have illustrated their findings, not only to share information but to find greater footing on the terrain we call biology: the science of life.
These models have taken on new urgency with the advent of cell biology, where subjects are even smaller than cells. “This is an invisible space,” Janet Iwasa, molecular visualization expert and Assistant Professor of Biochemistry at the U, reminds us. “Most molecules are smaller than the wavelength of light. These things are moving at a time scale that is not intuitive. When the study objects are so foreign, you have to rely on creative approaches to describe them.”
For Iwasa, those approaches involve scientifically accurate digital animations which have cracked open an entirely new way of viewing diverse molecular and cellular processes. Information-rich and visually compelling visualizations that capture current understanding is what this classically-trained biologist has made a name for herself with.
The need for reconsideration of the visual language that renders the invisible became urgent after a 2009 publication in Science of a much-cited article. The seminal paper posited that cellular structures called P granules are liquid droplets, and that they specify the future germline in a developing embryo through controlled dissolution and condensation. This paper ignited one of the hottest ‘trends’ in cell biology – the study of biological liquid condensates – and earned the lead authors numerous prizes, including, most recently, the prestigious Breakthrough Prize.
For Ofer Rog, Assistant Professor and Mario Capecchi Chair in the School of Biological Sciences, this revelation completely revised the interpretation of his experiments, but also brought with it “whole sets of biological issues.” The existence of crowding in the cell was one of them. No longer could he try to reduce the behavior of the chromosomes he was studying to properties of single molecules that make them up. “Rather,” says Rog, “we had to understand them as collective or ‘emergent’ behavior.”
With this new understanding, Rog felt “stuck” in his teaching and research with an old graphical language which “was really great for depicting things that are best understood as single objects, but not so great to describe how big clusters work together, to describe how molecules interact with each other much more loosely and much more dynamically.” The recognition of the flexibility and dynamics of cellular components led to the impulse to better honor that complexity graphically.
“I started looking at papers, and how uniform they were,” Rog says. “Papers that were clearly written with a lot of careful attention to details, with exquisite experiments and data, were using graphical models that were very simplistic, inadequate to really capture . . . our new understandings about biology. I started wondering, ‘How did people solve this in the past? Who should we talk to?’ It wasn’t super clear. So I went and talked to Janet.”
Powerful Renderings
They say the most dangerous thing one can do is to introduce one person to another. It’s a tongue-in-cheek caution, reminding us how conversations, then collaborations, then innovations start. So it was with Iwasa’s animation expertise which, as part of her Animation Lab at the University of Utah, has already animated many subjects, including the life-cycles of HIV and SARS-CoV-2. Now the lab is pairing its expertise with Rog’s condensate research.
“We have a lot of people, like Ofer,” says Iwasa, “who are educators and who have been using our animations for their courses. Condensate research is so new, compared to other big concepts in biology, that a lot of textbooks don’t even cover it. So, having some visual materials for educators who need an intuitive way to introduce these ideas to students was something we were thinking about.” Iwasa’s team had already interviewed undergraduate instructors to find out how they were teaching about condensates and what kinds of challenges they were facing.
And how were professors like Rog teaching about this new paradigm? Not easily, it turns out. The terrain was daunting. Intrigued, the Animation Lab began collaborating with Rog and other cell biologists to better illustrate condensates. “This new paradigm,” writes Rog and Iwasa of their collaboration, challenges “the 20th century textbook view of cellular compartmentalization.” Condensates, she says, seem to play important roles in cells’ normal functioning and in disease, and, naturally, these concepts are now making their way into undergraduate classrooms.”
Metaphors can be dangerous
Introducing two people is not the only dangerous thing to happen out there. There are implications of and uses for blending digital animation with biology and other sciences: representations–visual or verbal–are essential tools but at the same time impose biases. Because of simplification, “metaphors can be dangerous,” Iwasa concedes. “[P]eople don’t know how far they can carry them on a molecular level.”
The “language” of graphic representations, according to Rog, have tended to focus on single atomized cell components, and also incorporated implicit assumptions taken from our daily lives.
Iwasa agrees. Imagining the molecular space is “unintuitive, since it is unlike the air- and gravity-filled world we live in. What does a molecule experience being inside the cell? It’s just very different and hard to conceive. Some metaphors can be misleading. For example, there are proteins in the cell that move using a walking-like motion. Says Rog, “We walk in air, but when a molecule “walks,’ it’s the equivalent of us walking through Jell-O . . .”
“. . . Or walking in one of those children’s ball pits,” interjects Iwasa. “Except the balls are as big as you are, and you’re constantly bumping into everything, having to push things around.” The constant collisions, the extreme crowding: biologists know about these qualities, but because they don’t often depict that space, “it’s easy to forget and not to consider that, and that influences the types of experiments and the types of models we create.”
Illustrations did occasionally remind biologists of the crowded environment that occupies their objects of study. David Goodsell, a structural biologist and watercolor artist at the Scripps Research Institute in San Diego, is famous for his colorful illustrations of the interior of cells. These paintings are based on state-of-the-art knowledge of what is in the cell–what molecules exist in different sub-cellular compartments and what structures each of them adopts–but also capture the incredible complexity of the cell and, crucially, its crowdedness.
The new science of condensates relies on crowding for the ability of cellular structures to come together and fall apart. Rog, excitedly, returns to the human model and talks about “a thousand objects, like humans, in a crowded subway station, loosely associated” which, nevertheless, remain discrete individuals. How do those individuals behave separately? And how does that behavior change when they function as a collective?
New visual language and recent technological development promise to do a better job of depicting such complexity. Such representations continue to inform scientific discourse, as startling and revealing as 16th Century drawings brought to life through Vesalius’s magisterial bodies-in-motion.
The Workshop
Which leads us to the Re-Imagining a Cellular Space Occupied by Condensates symposium and workshop, borne out of the ready collaboration between Rog and Iwasa. While the Animation Lab’s initial foray into condensates was, in the beginning, educationally focused, that somewhat limited approach may now be at an inflection point.
“When Ofer and I talked,” says Iwasa, “we agreed that the research community had not yet reached any sort of consensus on how best to represent condensates. So our attempts to capture condensates by animation didn’t have a visual language to fall back on.”
Greater consensus may emerge at the symposium & workshop on October 11-13. Unlike the many traditional meetings dedicated to condensates, where scientists present and debate the minute details of their experiments, here scientists will interact with illustrators and other “tool builders,” to discuss the visual language of condensates.
While there is always a risk in illustration (including digital animation) of simplifying things too much and thus restricting future perceptions and scientific understanding, the symposium also pre-supposes that the conversation is essential. In short, the gathering promises to “daylight” how biologists represent a subcellular world in enabling as well as disabling ways, seeking “to build a community that will construct a visual language and new tools that will accurately capture the complexity of molecular condensates.” These representations will help generate experimentally-testable hypotheses, and will lead to the development of new techniques for scientific communication and teaching.
“One of the things that we realized,” says Rog, “is that challenges similar to the one we are facing now, in the condensate field, must have been figured out by other fields in the past, in biology and outside biology.” Symposium participants will include experts from diverse disciplines: about one-third of the participants are biologists, actively engaged in condensate research; one-third will be visualization and computation specialists—like watercolorist David Goodsell mentioned above—but also modeling experts, data visualization specialists, and molecular animators.
The final one-third will come from fields that are not commonly engaged with molecular biology but that have long been thinking about space and ways to represent it. This last group includes software and virtual reality developers and academics in architecture and history.
The symposium will take place at the Crocker Science Center at the University of Utah, on October 11, 2022, 9 AM to 5 PM, and is open to the public. It will be followed by a two-day workshop (by invitation only).
By David Pace. First published @ biology.utah.edu
Golden Goose 2022
Golden Goose Award
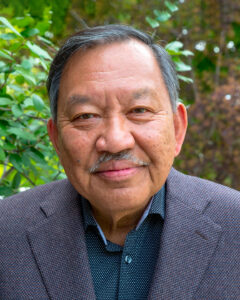
Baldomero "Toto" Olivera
A side hustle that transformed neuroscience.
As scientists working in the Philippines in the 1970s, biochemists Baldomero Olivera and Lourdes Cruz, professor emeritus of the University of the Philippines Diliman, found it tough to get hold of the right supplies for DNA research.
“We had to find something to do that didn’t require fancy equipment because we had none,” said Olivera, a distinguished professor at The University of Utah’s School of Biological Sciences, in a video produced for the Golden Goose awards.
Olivera and Cruz came up with what they hoped would be a fruitful side project. Cone snails are commonplace in the Philippines, and they had always fascinated Olivera, who had collected shells as a child. The pair decided to research the nature of the venom that the snails used to paralyze their tiny fish prey.
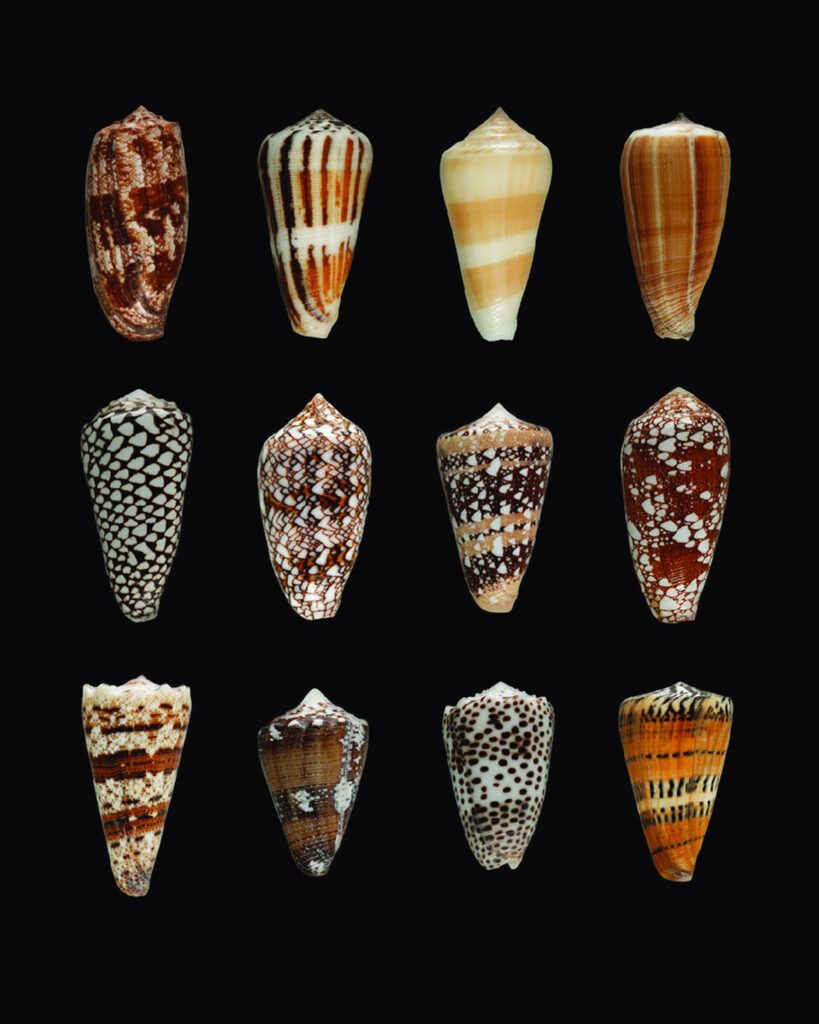
Cone Snail Shells
The team discovered the bioactive compounds in the venom were tiny proteins known as peptides. After moving to the US and teaming up with University of Utah grad students Dr. Michael McIntosh and the late Craig T. Clark, Olivera and Cruz learned that some of the venom peptides reacted differently in mice than in fish and frogs. It turned out in mammals the compounds were involved in the sensation of pain, rather than muscle paralysis.
“There was this incredible gold mine of compounds,” said McIntosh in the video. He is now a professor and director of research of psychiatry in the School of Biological Sciences at The University of Utah.
On September 14, 2022, the American Association for the Advancement of Science (AAAS), the world’s largest multidisciplinary scientific society, hosted the 11th annual Golden Goose Award ceremony, a celebration of federally funded research that unexpectedly benefits society. AAAS awarded University of Utah research of a non-opioid pain reliever, hidden in the venom of tiny cone snails, which greatly decreases pain for patients with chronic illnesses while helping scientists develop new ways to map the body’s nervous system. As undergraduate researchers, Craig Clark (in memoriam) and J. Michael McIntosh, now a professor of psychiatry at the U, isolated a compound that eventually led to an approved non-opioid pain killer. Baldomero M. Olivera, Distinguished Professor in the School of Biological Sciences, and Lourdes J. Cruz, then faculty of biology at the U and now Professor Emeritus at the University of the Philippines, supervised the research. The award recognizes all four individuals.
The Golden Goose Award spotlights scientific research that may have appeared obscure, sounded funny, or for which the results were unforeseen at the outset but ultimately, and often serendipitously, led to breakthroughs. This year, the award comes on the heels of the U.S. Congress passing and President Biden signing the bipartisan and historic CHIPS and Science Act. This new law reauthorizes key federal agencies whose projects will propel discovery, build on our strengths, and show what American investment, intellect, ingenuity and risk-taking can accomplish — precisely the type of innovation the Golden Goose Award honors.
U.S. Representative Jim Cooper (D-TN), often referred to as “Father Goose,” will retire from Congress at the end of this term. He conceived of the award as a strong counterpoint to criticisms of basic research as wasteful federal spending, such as the late Sen. William Proxmire’s (D-WI) Golden Fleece Award, leading to a coalition of business, university, and scientific organizations establishing the award in 2012. Thanks to his legacy, the award will continue to elevate the importance of recognizing basic science that ultimately improves people’s quality of life.
“The Golden Goose Award reminds us that potential discoveries could be hidden in every corner and illustrates the benefits of investing in basic research to propel innovation,” said Sudip S. Parikh, chief executive officer at AAAS and executive publisher of the Science family of journals. “AAAS is honored to elevate this important work since the award’s inception, and we thank Representative Cooper for his tireless leadership and dedicated support to this award and the scientific community.”
Tiny snail, big impact
In the 1970s, Olivera and collaborator Cruz were interested in the deadly venom used by cone snails, marine creatures native to the Philippines. When Olivera moved to the U, his focus shifted to other areas, but he kept the cone snail venom as a side project. In 1979 he assigned two undergraduate researchers the task of isolating the venom’s components and testing their impacts on mice. Craig Clark, a sophomore biology major, and McIntosh, a 19-year-old who just graduated high school, discovered something unexpected—a compound they named “shaker peptide” blocked calcium channels in the mice, which are the nerve’s ability to communicate with the rest of the body. Later, they found that the shaker peptide specifically targeted the channels related to pain in mammals and is 1,000 times as powerful as morphine. McIntosh is now a professor of psychiatry at the U with his own lab and thirty years later, continues to work with Olivera to explore the therapeutic potential of cone snail venom that has one of the most promising non-opioid alternatives to manage pain. One compound become an FDA-approved painkiller.
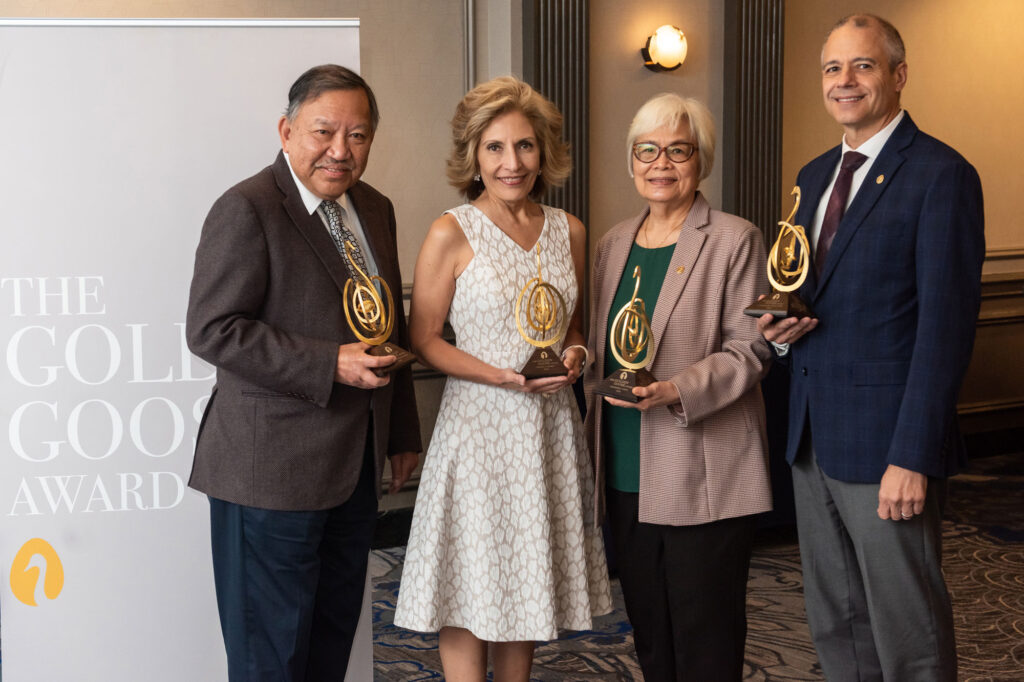
2022 Golden Goose Awards Ceremony
The student project of Clark and McIntosh is part of a long tradition of undergraduate research in the U’s College of Science. Fifty years ago, K. Gordon Lark, the first chair of the Department of Biology at the U, started an initiative to support undergrad research opportunities in faculty laboratories, an initiative that led to recruiting biology undergraduates such as Clark and McIntosh. The College of Science is expanding his legacy under a newly created Science Research Initiative, which provides most U science undergraduates with a unique opportunity to pursue their own independent research projects.
2022 Golden Goose Awardees:
Craig T. Clark (in memoriam), Lourdes J. Cruz (University of the Philippines), J. Michael McIntosh (University of Utah; George E. Wahlen VA Medical Center), and Baldomero Marquez Olivera (University of Utah)
Tiny Snail, Big Impact: Cone Snail Venom Eases Pain and Injects New Energy into Neuroscience
Impeded by supply chain issues while conducting DNA research in the Philippines, Lourdes Cruz and Baldomero Olivera began examining cone snails, a group of highly venomous sea mollusks which happened to be in abundant supply along the country’s coastal waters. Several decades and countless airline miles later, and with the help of then-undergraduate students Craig Clark and Michael McIntosh, the team discovered the raw material for a non-opioid pain reliever and a powerful new tool for studying the central nervous system, all hidden in the cone snail’s potent venom
Ron Kurtz (RxSight), Tibor Juhasz (ViaLase), Detao Du (Rayz Technologies), Gerard Mourou (Ecole Polytechnique), and Donna Strickland (University of Waterloo)
How a Lab Incident Led to Better Eye Surgery for Millions of People
Nearly 30 years ago, a graduate student at the University of Michigan’s Center for Ultrafast Optical Science (CUOS) experienced an accidental laser injury to his eye. Fortunately, his vision was not severely affected. However, the observation of the very precise and perfectly circular damage produced by the laser led to a collaboration. Eight years later, that group of researchers developed of a bladeless approach to corrective eye surgery. The new procedure, also known as bladeless LASIK, uses a femtosecond laser rather than a precision scalpel cut into the human cornea before it is reshaped to improve the patient’s vision.
Manu Prakash (Stanford University) and Jim Cybulski (Foldscope Instruments Inc.)
Foldscopes and Frugal Science: Paper Microscopes Make Science Accessible
While researching in remote areas of India and Thailand, a technical challenge piqued Manu Prakash’s curiosity. In certain areas of the world, transport, training, and maintenance barriers can make state-of-the-art microscopes inaccessible. Prakash found a potential solution in a decidedly un-technical material: paper. Using principles of origami applied to printer paper, matchboxes, and file folders, Prakash and graduate student Jim Cybulski designed a paper microscope known as the Foldscope that can achieve powerful magnification with materials that cost less than $1 to manufacture. Today, just over a decade later, two million Foldscopes have been distributed in over 160 countries and have been used to diagnose infectious diseases, diagnose new species, and identify fake drugs, among many other applications.
>> HOME <<
At-Risk Forests
At-Risk Forests
Global analysis identifies at-risk forests.
Forests are engaged in a delicate, deadly dance with climate change, hosting abundant biodiversity and sucking carbon dioxide out of the air with billions of leafy straws. They can be a part of the climate solution as long as global warming, with its droughts, wildfires and ecosystem shifts, doesn’t kill them first.
In a study published in Science, William Anderegg, the inaugural director of the University of Utah’s Wilkes Center for Climate Science and Policy, and colleagues quantify the risk to forests from climate change along three dimensions: carbon storage, biodiversity and forest loss from disturbance, such as fire or drought. The results show forests in some regions experiencing clear and consistent risks. In other regions, the risk profile is less clear, because different approaches that account for disparate aspects of climate risk yield diverging answers.
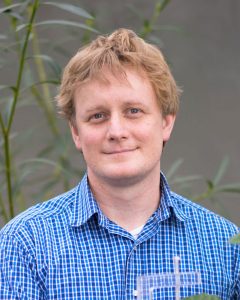
William Anderegg
“Large uncertainty in most regions highlights that there's a lot more scientific study that's urgently needed.”
An international team
Anderegg assembled a team including researchers from the United Kingdom, Germany, Portugal and Sweden.
“I had met some of these folks before,” he says, “and had read many of their papers. In undertaking a large, synthetic analysis like this, I contacted them to ask if they wanted to be involved in a global analysis and provide their expertise and data.”
Their task was formidable –assess climate risks to the world’s forests, which span continents and climes and host tremendous biodiversity while storing an immense amount of carbon. Researchers had previously attempted to quantify risks to forests using vegetation models, relationships between climate and forest attributes and climate effects on forest loss.
“These approaches have different inherent strengths and weaknesses,” the team writes, “but a synthesis of approaches at a global scale is lacking.” Each of the previous approaches investigated one dimension of climate risk: carbon storage, biodiversity, and risk of forest loss. For their new analysis, the team went after all three.
Three dimensions of risk
“These dimensions of risk are all important and, in many cases, complementary. They capture different aspects of forests resilience or vulnerability,” Anderegg says.
- Carbon storage: Forests absorb about a quarter of the carbon dioxide that’s emitted into the atmosphere, so they play a critically important role in buffering the planet from the effects of rising atmospheric carbon dioxide. The team leveraged output from dozens of different climate models and vegetation models simulating how different plant and tree types respond to different climates. They then compared the recent past climate (1995-2014) with the end of the 21st century (2081-2100) in scenarios of both high and low carbon emissions. On average, the models showed global gains in carbon storage by the end of the century, although with large disagreements and uncertainty across the different climate-vegetation models. But zooming in to regional forests and taking into account models that forecast carbon loss and changes in vegetation, the researchers found higher risk of carbon loss in southern boreal (just south of the Arctic) forests and the drier regions of the Amazon and African tropics.
- Biodiversity: Unsurprisingly, the researchers found that the highest risk of ecosystems shifting from one “life zone” to another due to climate change could be found at the current boundaries of biomes – at the current transition between temperate and boreal forests, for example. The models the researchers worked from described changes in ecosystems as a whole and not species individually, but the results suggested that forests of the boreal regions and western North America faced the greatest risk of biodiversity loss.
- Disturbance: Finally, the authors looked at the risk of “stand-replacing disturbances,” or events like drought, fire or insect damage that could wipe out swaths of forest. Using satellite data and observations of stand-replacing disturbances between 2002 and 2014, the researchers then forecast into the future using projected future temperatures and precipitation to see how much more frequent these events might become. The boreal forests, again, face high risk under these conditions, as well as the tropics.
“Forests store an immense amount of carbon and slow the pace of climate change,” Anderegg says. “They harbor the vast majority of Earth's biodiversity. And they can be quite vulnerable to disturbances like severe fire or drought. Thus, it's important to consider each of these aspects and dimensions when thinking about the future of Earth's forests in a rapidly changing climate.”
Future needs
Anderegg was surprised that the spatial patterns of high risk didn’t overlap more across the different dimensions.
“They capture different aspects of forests' responses,” he says, “so they wouldn't likely be identical, but I did expect some similar patterns and correlations.”
Models can only be as good as the basis of scientific understanding and data on which they’re built and this study, the researchers write, exposes significant understanding and data gaps that may contribute to the inconsistent results. Global models of biodiversity, for example, don’t incorporate dynamics of growth and mortality or include the effects of rising CO2 directly on species. And models of forest disturbance don’t include regrowth or species turnover.
“If forests are tapped to play an important role in climate mitigation,” the authors write, “an enormous scientific effort is needed to better shed light on when and where forests will be resilient to climate change in the 21st century.”
Key next steps, Anderegg says, are improving models of forest disturbance, studying the resilience of forests after disturbance, and improving large-scale ecosystem models.
The recently-launched Wilkes Center for Climate Science and Policy at the University of Utah aims to provide cutting-edge science and tools for decision-makers in the US and across the globe. For this study, the authors built a visualization tool of the results for stakeholders and decision-makers.
Despite uncertainty in the results, western North America seems to have a consistently high risk to forests. Preserving these forests, he says, requires action.
“First we have to realize that the quicker we tackle climate change, the lower the risks in the West will be,” Anderegg says. “Second, we can start to plan for increasing risk and manage forests to reduce risk, like fires.”
Find the full study here.
by Paul Gabrielsen, first published in @theU.